This article explores into the historical evolution of structural engineering forms, meticulously unraveling the intricate tapestry of milestones that have not only shaped the discipline but have also woven together the very fabric of human civilization.

Introduction
The roots of structural engineering can be traced back to the ancient civilizations, where early engineers developed innovative solutions to construct impressive structures with limited resources. Throughout history, civilizations have consistently needed structures for habitation, religious practices, military purposes, and communal pride. Our understanding of ancient societies like the Egyptians, Greeks, Romans, and others largely stems from the analysis of their architectural heritage. Their architects and engineers faced limitations imposed by the available materials and technology of the time.
Egyptian constructions predominantly relied on mud bricks and stone due to the scarcity of timber. Structural form was mostly confined to walls, columns, and beams, with elements characterized by massive proportions.
The Greek’s structures predominantly consisted of stone, showcasing sophisticated designs and remarkable elegance. Despite their advanced architectural prowess, their technological capabilities were constrained, with trusses being an unfamiliar concept and their constructions featuring relatively modest spans.
Comparatively, the Romans were advanced in the art of building. With the use of concrete, they successfully covered spans of roofs reaching up to 30m, and a remarkable example is the 43m-wide dome of the Pantheon (Figure 1). They possessed a comprehensive understanding of triangulation, allowing Roman carpenters to roof over considerable distances.
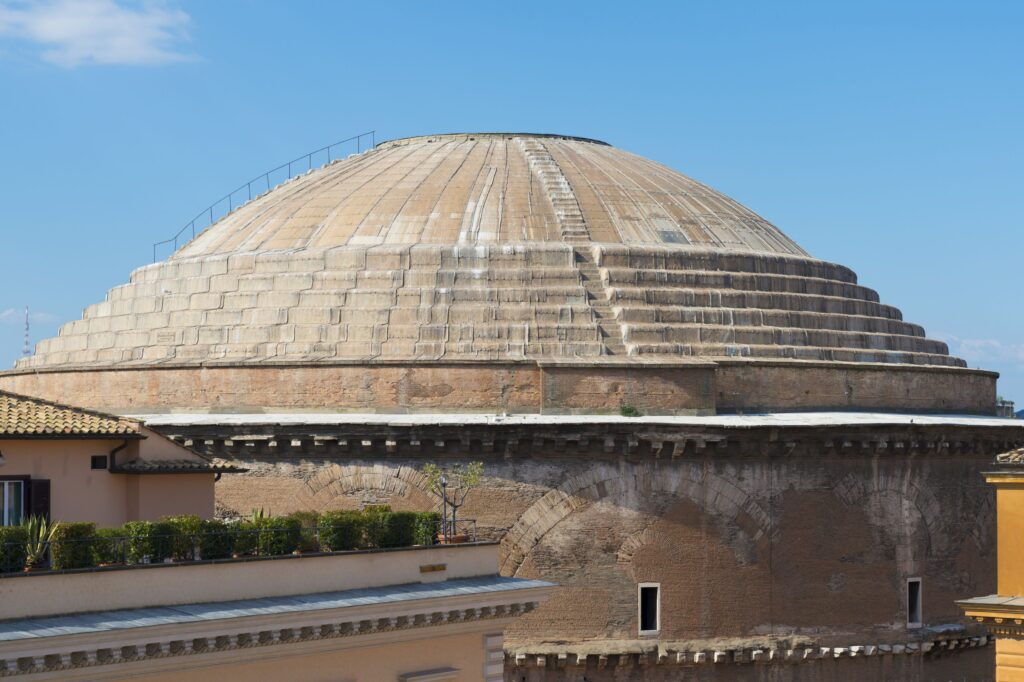
Today is a testimony of how far we have come. Structural engineering, as a discipline has been at the fore in shaping the built environment throughout history. The evolution of structural engineering forms is a fascinating journey that reflects advancements in technology, changes in architectural styles, and the constant pursuit of innovation to create safer, more efficient, and aesthetically pleasing structures. From the ancient civilizations to the present day, the development of structural engineering has been marked by transformative moments and breakthroughs.
This article meticulously unravels the intricate tapestry of milestones that have shaped the discipline by exploring the historical evolution of structural engineering forms across four significant periods:
- Medieval – Modern Times
- 18th and 19th century.
- First half of the 20th century.
- Second half of the 20th century.
Medieval – Modern Times
The growth of structural form from medieval to modern times progressed at a relatively slow pace. Many structures were characterized by massiveness, often resembling fortifications. Palaces prioritized grandeur with an emphasis on architecture over engineering considerations. The prevalent materials, such as brick, stone, and timber, remained largely unchanged. However, two significant changes in structural form emerged in connection with the expansion of cathedral architecture in stone.
Firstly, there was an evolution in the shape of arches from the ‘Roman round’ to the ‘Norman pointed,’ accompanied by a gradual reduction in solidity. The pinnacle of cathedral elegance during the Gothic perpendicular style around the 15th century showcased advanced techniques in managing load paths and ensuring stability. Notably, there were considerable skills demonstrated in enclosing space through the use of vaulted roofs (see Figure 2).
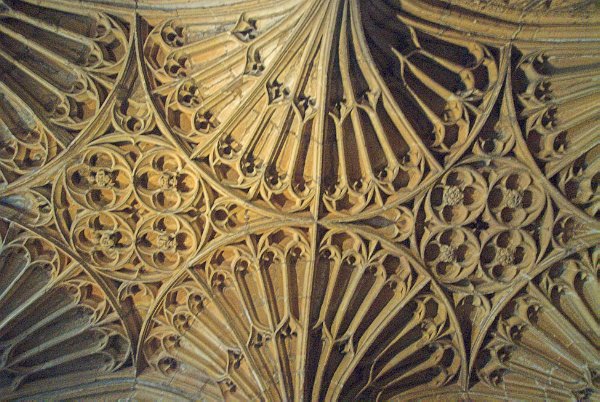
The second major innovation resulted from the expertise of carpenters in developing timber trusses and the frame form. The potential of this innovation is evident in still-existing timber roofs, such as the trusses in the St Rhychwyn’s church (12th century) (Figure 3).
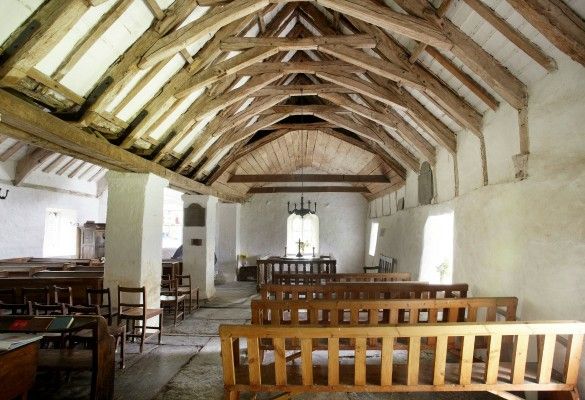
Note:
- The diagonal struts do not meet at a point at mid-span. This induces local bending in the chord member under non-symmetric load.
- Also, the truss can act to share any horizontal forces between the two supports and resist them by the bending of the bottom chord and supporting columns.
This technique continued to evolve, leading to the development of English hammer beam roofs in the later part of the 14th century. An exemplary illustration is the impressive Westminster Hall, boasting a remarkable span of 21m – the longest in all of Europe during that era (see Figure 4).
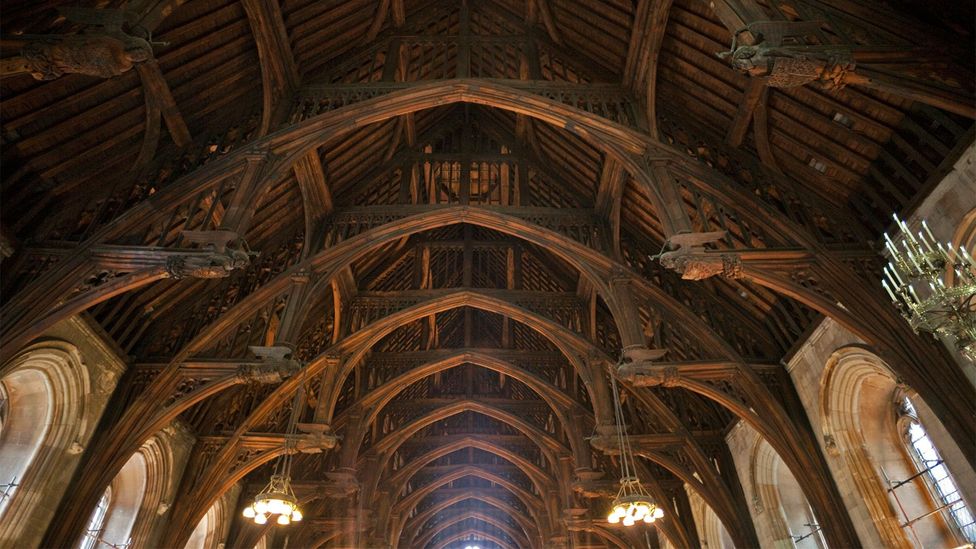
An examination of structures from the medieval periods points to the fact that masons and timber craftsmen must have possessed an exceptional understanding of structural engineering interventions required for stabilization. Masonry structures, dependent on balancing gravity forces, occasionally received assistance, as seen at Salisbury Cathedral (1220–1258), where the UK’s tallest spire (123m) was stabilized by pre-stressing its masonry along with a rod and turnbuckle.
18th & 19th Century
Obvious reasons drove significant transformations in structural forms during the 19th century. Firstly, a substantial increase in urban population created an overwhelming demand for expanded infrastructure, all within the context of rising living standards. Secondly, the Industrial Revolution necessitated the establishment of factories and improved transportation systems to facilitate the global distribution of goods, giving rise to complex structures. Also, the railway boom and canal building era, which preceded it, led to the construction of bridges, viaducts, railway sheds, and docks.
The revolution itself brought about cheap power generation and the economical production of iron, paving the way for the extensive use of steel as a structural material. The world’s oldest surviving railway bridge, the stone Causey Arch near Newcastle (1725), spanning 30m, adopted its form from Roman precedents. Subsequent changes ensued, marked by the construction of the world’s first iron bridge in 1779 at Coal Brookdale, Shropshire, also spanning 30m (Figure 5).
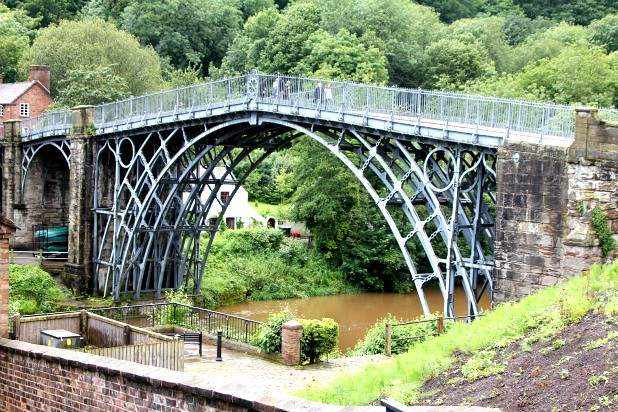
Note:
- This structure is pinned at mid-span, so it is just a simple three pinned arch
- The rings make it look complicated, but they only serve the purpose of transferring the deck forces to the arch.
Development progressed rapidly in the 19th and 20th century and subsequent innovations swiftly followed suit. The Myste cast iron bridge (1823-26) stood out not only for its impressive span but also for introducing ingenious concepts of prefabrication. Advancements in construction were imperative for spanning longer distances and accommodating heavier loads.
A significant breakthrough occurred in the early 1800s with the development of suspension bridges, exemplified by the renowned Menai Suspension Bridge (1826). Thomas Telford, in its construction, utilized chains; however, the later invention of steel wire in the 1830s facilitated the construction of longer suspension bridges in the United States, laying the groundwork for contemporary bridges.
Simultaneously, Robert Stephenson introduced the box girder for railways through the Britannia Bridge (1845–1850), featuring an impressive span of 70m (Figure 6). These remarkable developments hinged on the ready availability of metal as a construction material, supported by industrial processes for shaping and the joining technology of hot riveting.
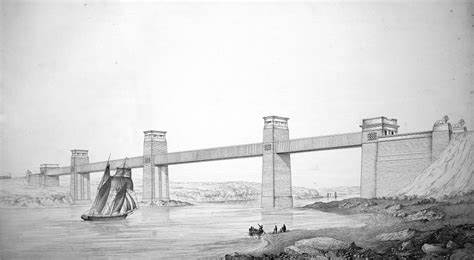
Generally speaking, the 18th and 19th centuries were characterized by longer spans and the introduction of metal, specifically steel, which fundamentally defined this period. Steel provided unprecedented opportunities for constructing larger and taller structures, meeting the escalating demand for infrastructure as the century neared its end. During this era, the construction of high-rise buildings emerged, incorporating exclusively steel frames. Until the 1900s, the utilization of concrete for framing significantly lagged behind steel, although it found application in floors and foundations.
Despite the discovery of Portland cement in 1824, the most impactful form of concrete, reinforced concrete, did not come to fruition until almost a century later in 1892. The 15-storey Ingall building, constructed around 1903, claiming the title for the first reinforced concrete building.
Subsequently, the development of structural forms conveniently paralleled the history of the concrete institute, established in 1908, which later underwent a rebranding as The Institution of Structural Engineers in 1922.
First half of 20th Century
The early 20th century saw the rise of modernism, characterized by a departure from historical architectural styles and a focus on functional design. The first half of the 20th century witnessed substantial urban development, momentarily hampered by the Great Depression starting around 19301.
At the outset of this era, steel was readily accessible, firmly establishing the trend of framing buildings instead of relying on load-bearing masonry for support. Although high-rise steel-framed buildings were being erected in the United States, comparable structures in the UK were constrained by Building Regulations. The Ritz hotel in London is often acknowledged as the first fully steel-framed building structure in Britain, completed in 1904 with a height of merely eight stories (Figure 7). In contrast, across the Atlantic, the Empire State Building in New York reached an impressive 102 stories (381m) by 1931.
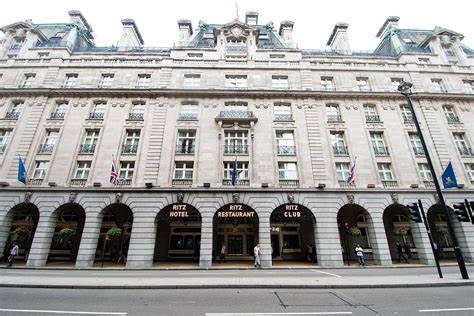
Framed structures provided architects with numerous advantages, allowing for greater freedom of expression, particularly by eliminating the need for internal support walls. They also facilitated speedy construction and cost reductions, both influential factors in shaping structural form. During this period, some building rates achieved were truly remarkable, exemplified by the British Empire Stadium at Wembley, seating 125,000 people, which was constructed in just 12 months in 1924, leveraging modularization.
The UK saw the construction of tall concrete structures, most notably the Liver Building in Liverpool (1911), towering at a height of 51m. Concrete gained prominence due to its competitiveness, offering the opportunity to construct entire structures, including floors and supports, using a single material.
This proliferation of systems, combined with a desire to realize the benefits of fire protection, served as the driving force behind the establishment of The Concrete Institute. Industry demands for power stations and factories played a crucial role, with the advent of new materials and enhanced transportation and fabrication possibilities contributing to iconic steel builds. Battersea Power Station (Figure 8), completed in 1933, utilized thousands of tonnes of steel. While internally framed with steel, its exterior walls consisted of brick, retaining its status as the largest brick building in Europe. For factories, a variety of latticed steel frames emerged, often covered with corrugated galvanized sheets or an economical composite material— asbestos-reinforced cement sheets (no longer in use). Evidently, minimal attention was paid to aesthetics or insulation.
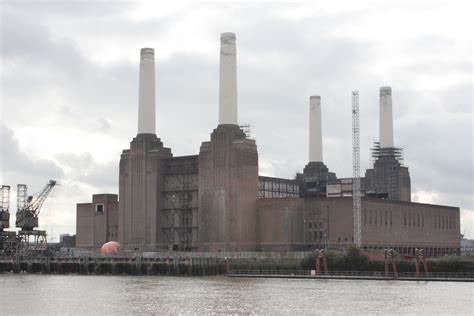
With respect to bridges, the early 20th century also witnessed several notable achievements, with the Tyne Bridge of 1928 (7 tonnes) and a 162m span standing out as a prototype for a larger counterpart—the Sydney Harbour Bridge, completed in 1933 (refer to Figure 9).
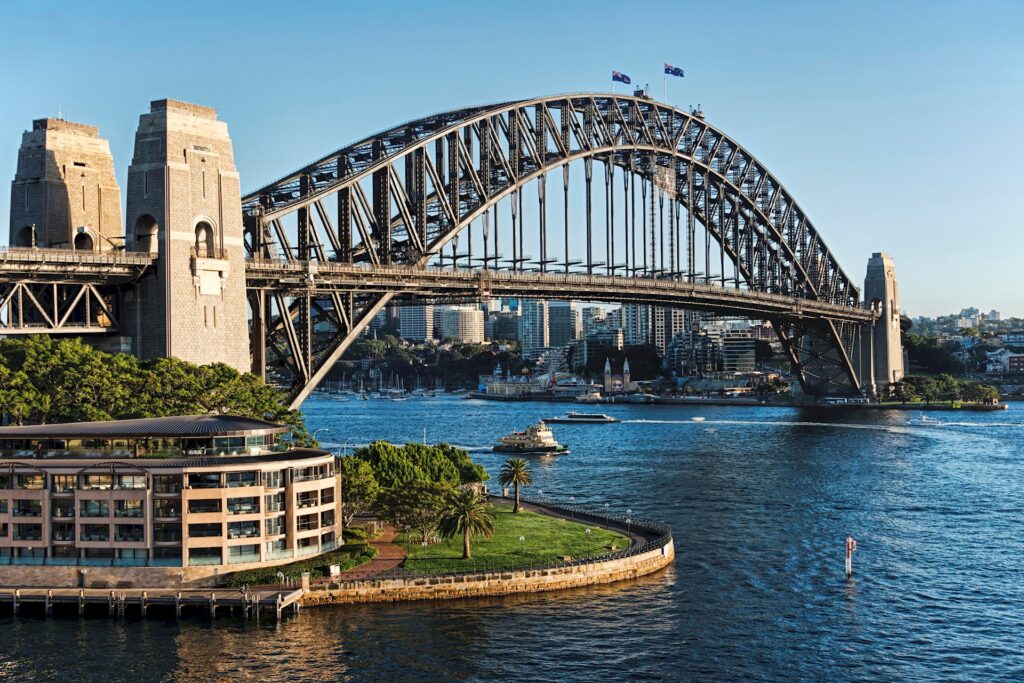
Note that this is similar to St Pancras except that:
- The arch truss is much deeper and therefore stiffer
- There is a continuous, slender deck in the middle, which is subject to local loading.
- And because the arch is much stiffer than the deck, it will carry most of the bending and shear associated with this local loading, as well as the compression from the arch action.
Several factors can be attributed to the changes in this era. Firstly, the construction industry received a steady supply of fundamental modern construction materials, presenting unprecedented opportunities. Concurrently, there were advancements in calculation techniques, instilling engineers with confidence in designing structures scientifically, although these techniques were not infallible. Less overtly, there was an increase in the standardization of parts, expanding their availability, coupled with the development of mechanical equipment—all enhancing production and efficiency within a maturing construction industry.
Second half of 20th Century
After the Second World War, various sectors, including construction, faced significant setbacks due to severe material shortages and a scarcity of skilled labor. Despite the challenges, the widespread destruction in Europe sparked a substantial demand for reconstruction, leading to the restoration of infrastructure, housing for millions, and the rebuilding of economies through modern factories. Societal pressures, driven by a strong aversion to a return to the poverty-stricken pre-war existence, compelled governments to advocate for the creation of entirely new, clean, and healthy cities. In the UK, the national sentiment was symbolized at the 1951 Festival of Britain, featuring notable structures like the Dome of Discovery and the futuristic Skylon (Figure 10).
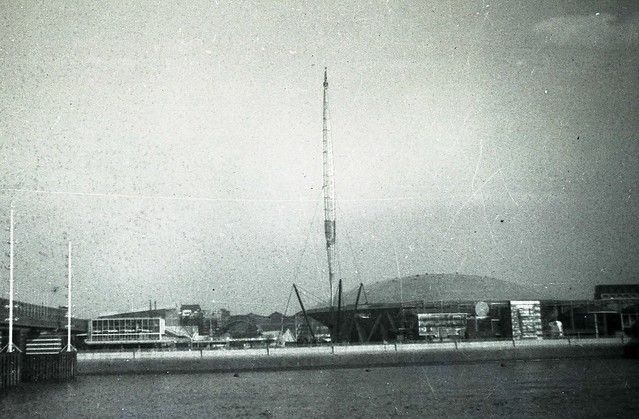
Note:
- The loading system is well triangulated, in principle ensuring stability of the structure under gravity and horizontal loads.
- There needs to be some minimal pre-stress under gravity loading (as shown on the right) to enable that the geometry of the cables does not change significantly.
- In fact, the hangers were pre-stressed substantially to improve geometric consistency.
Albeit there was no significant change in structural form relative to early part of the 20th century, there was however significant changes in how structures were designed. The conditions existing after the war strongly influenced the development of structural form in the early part of the century. Shortages in materials acted as a catalyst, driving the push for structural efficiency and fostering advancements in various technologies. The pressing demand for housing and the urgency for rapid construction played pivotal roles in incentivizing the development of system building for residential, educational, and healthcare structures. The vision was to manufacture high-quality components in factories and assemble them on-site, addressing the shortages in skilled labour.
These demands led to the development of codes of practices; standardization of structural steel sections and steel connections; concrete development in the form of reinforced concrete; pre-stressing and precast; significant development in masonry and timber, advances in geotechnics and foundation engineering; and emphasis on research and trade organizations.
It is this trajectory of innovation, emphasizing cost and reduction in material use and construction speed, that has persisted to the present day. The advent of computers also further propelled this drive, influencing information handling, quality control, automation, and numerically controlled machines.
Conclusion
Structural engineering forms have evolved throughout history, driven by a relentless pursuit of innovation and efficiency in design. From the medieval Gothic cathedrals to modern skyscrapers, each era has woven a unique thread into the fabric of structural engineering. Technology, sustainability, and contemporary architectural needs continually mold the field. Thus, the journey of structural engineering can only be said to underscore human creativity and resilience.
Also See: Top 7 Innovative Trends in Structural Engineering
Sources & Citation
- Mann, A.P (2016) “Historical Development of Structural Forms” Essential Knowledge Series. The Institution of Structural Engineers, Essential Knowledge Series Note 3. Available at: IstructE.org/resources/essential-knowledge-series (Assessed: Jan 2024 (Assessed: Jan 2024).
- Mann, A.P. “The historical development of structural theories and methods of analysis — context of modern computer analysis” The Institution of Structural Engineers – Essential Knowledge Text No. 4 [online] Available at: IstructE.org/resources/essential-knowledge-series (Assessed: Jan 2024