This article presents the design of embedded retaining walls – a wall in which resistance to active lateral earth pressure is mainly resisted by the passive pressure generated at the toe side of the wall.
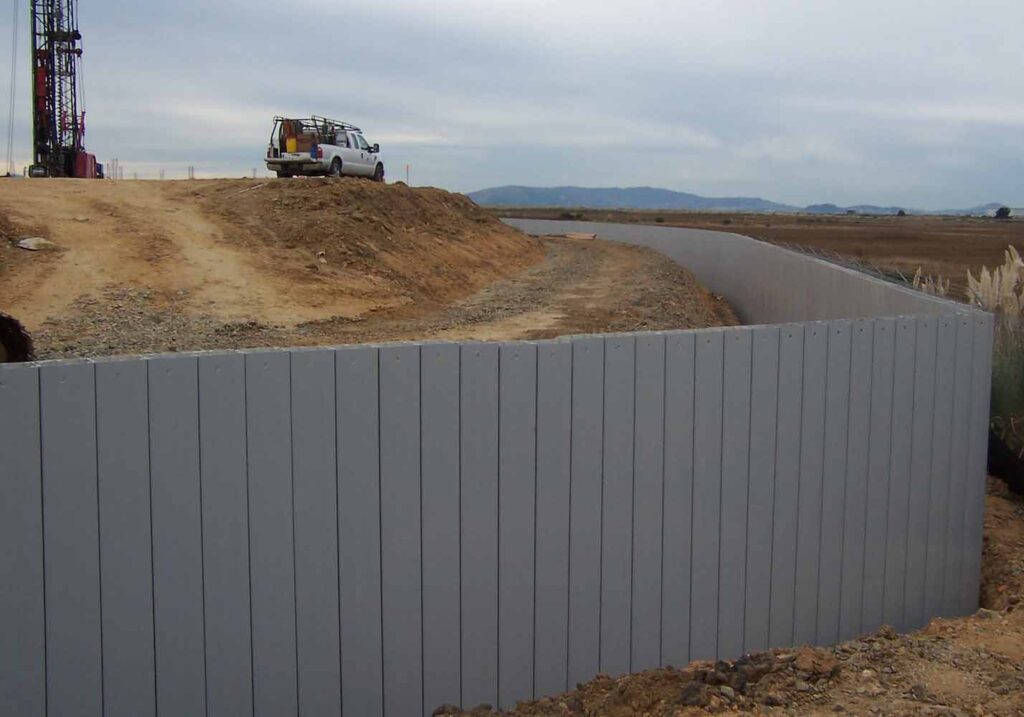
Embedded retaining walls, like all other retaining wall structures are designed to support soil and prevent landslides. They are often used in construction sites, highways, and residential developments where space is limited, and the risk of soil movement is high. The design of these walls requires careful consideration of the forces exerted by the soil and any external loads to ensure stability and durability.
These walls differ from conventional retaining walls (See: Retaining Walls Construction Methods) in that they are anchored deep into the ground, hence the term “embedded.” This depth provides the requisite stability, making them ideal for supporting deep excavations or retaining soil on steep slopes. Embedded retaining walls rely on the passive pressure generated at the front face of the wall and the shear and bending capacity of the walls material itself to remain stable. Thus, in designing an embedded retaining wall, the depth of embedment is crucial to their effectiveness, as it helps resist overturning and sliding forces.
They are very suitable for very deep excavation in excess of 10m, and since they require no prior excavation before installation, they’re very suitable for restricted sites. They are typically used for most temporary works, caisson foundations, bridge pier construction as well as many permanent installations.
The following sections will delve into the types of embedded retaining walls and the various aspects of designing an embedded retaining wall, including a fully worked example to illustrate the process.
Types of Embedded Retaining Walls
Sheet Pile Retaining Walls
Sheet piles (figure 1) are the most basic types of embedded retaining walls. They are made from profiled steel sheets connected together via an interlock that runs along the edge of each sheet. The profiled steel sheets during construction are driven into the ground via the use of a mechanical hammer.
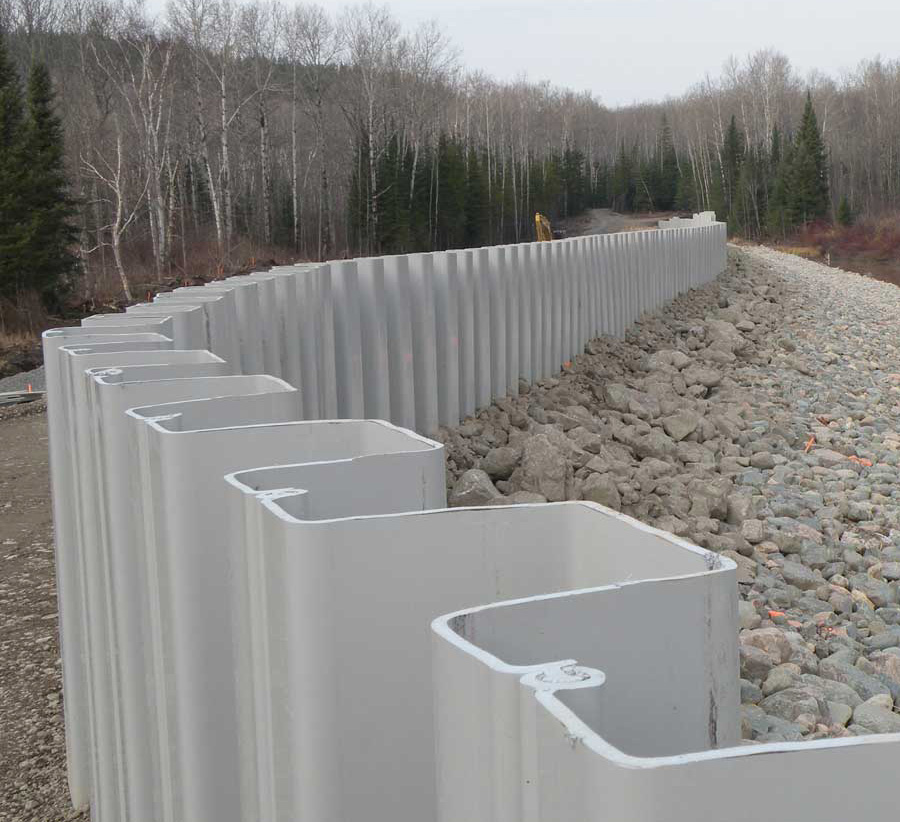
Sheet piles are generally used to retain clay, sand and silt soils with mostly no hard inclusion such as boulders or existing substructures.
Contiguous Bored Piled Retaining Walls
Contiguous bored piles walls are made of pile elements referred to as ‘contigs,’ dug and cast into the ground with a small gap of 50-150mm between each pile (figure 2). This category of embedded retaining wall is only suitable for retaining non-granular materials. Granular soils such as sand and gravel tend to fall through the gaps between the piles. In the same vein, they cannot retain water, thus they are only utilized in places where the water table is below the retained height.
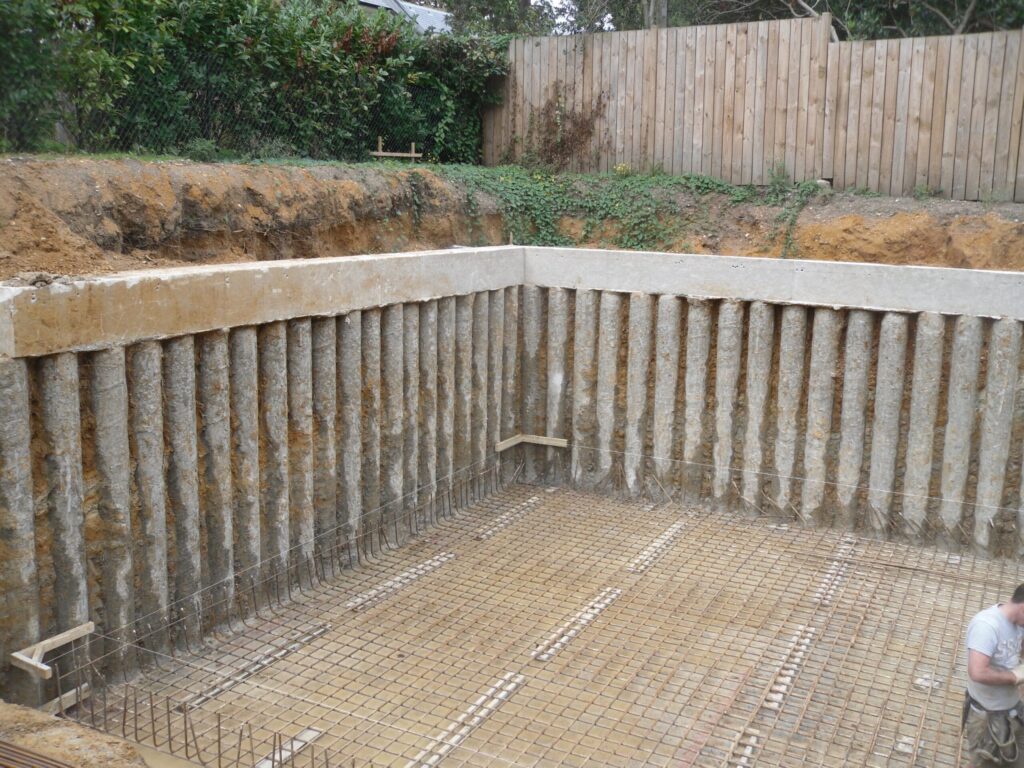
Secant Bored Piled Retaining Walls
The secant bored piled wall is an extension of the contiguous piled wall, with the only exception being the absence of the gaps between the piles. Here the piles are divided into primary and secondary (figure 3). The primary piles are installed first with the secondary piles overlapping the first. What this does is create a wall that is capable of resisting water penetration.
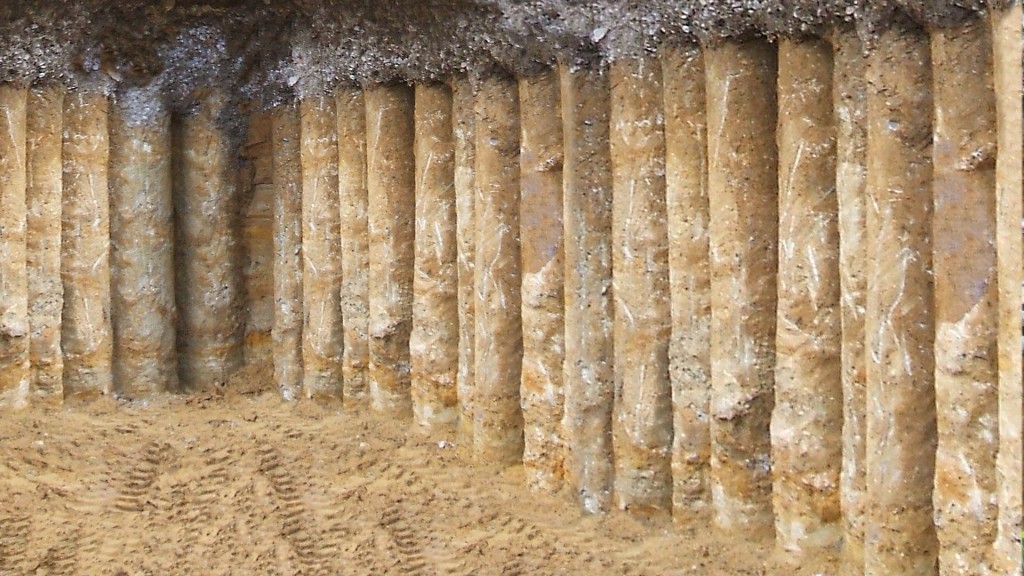
In contrast to the contigs, secant bored piled walls can be installed into almost any soil and can be placed even where there is a high-water table.
King-post Piled Retaining Walls
King post piled retaining walls, also known as soldier walls consist of a series of steel posts that are either driven into the ground or cast in place into an excavated hole (figure 4). The posts are typically spaced in the range of 1-3m apart and the gap formed between them filled with spanning panels which are generally made up of precast elements.
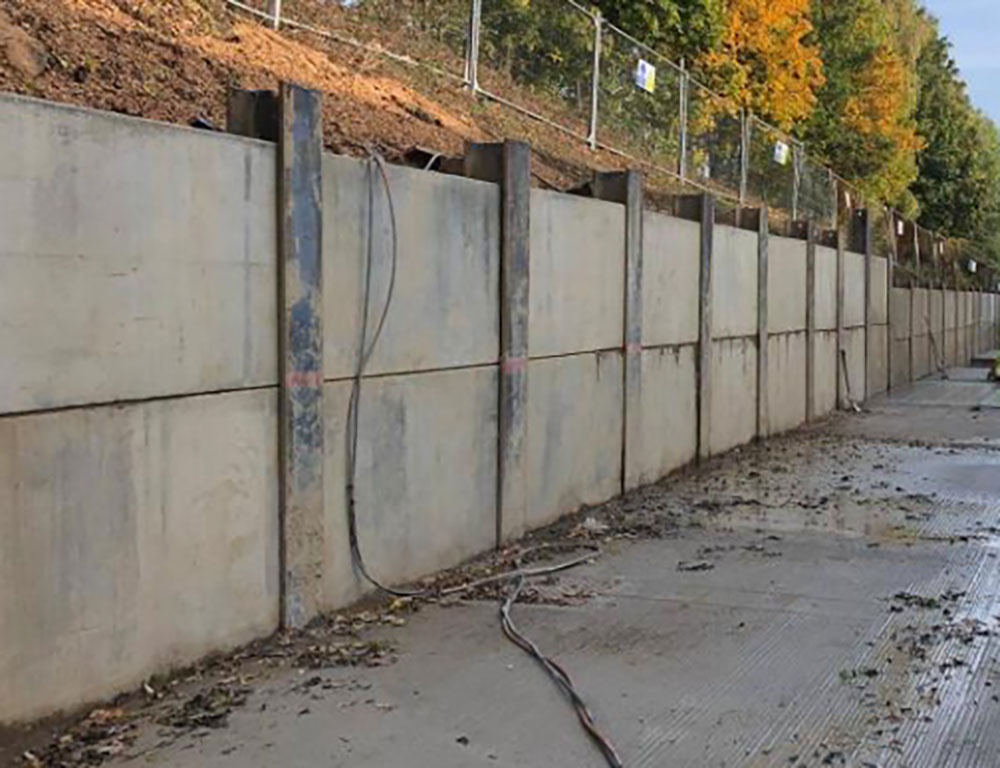
They are typically used to retain clay and granular soils and where the water table is below the required retained height.
Diaphragm Retaining Walls
Diaphragm walls (figure 5) are formed by excavating a segment of soil and replacing it with a fluid, after which a reinforcement cage is erected in the excavation and concrete poured in. As the concrete is poured into the excavation, the concrete displaces the liquid. Diaphragm walls are at the pinnacle of retaining walls construction, by virtue of being able to retain significantly more soil than any other retaining wall type. They can be up to 1500mm thick and can retain soil up to a depth of 120m.
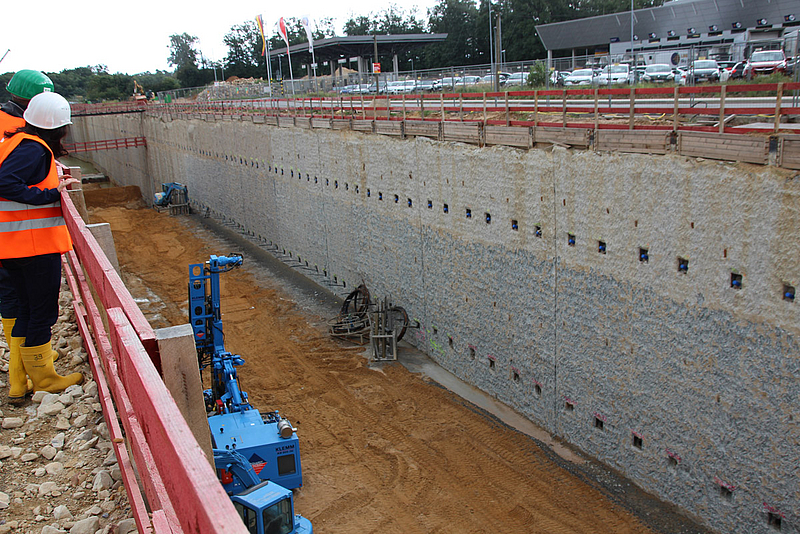
Diaphragm walls can be installed into almost all soil types irrespective of the position of the water table. They are able to retain water pressures provided water bar seals are installed across each segment of the diaphragms.
Principle of Embedded Wall Design
The underlying principle to remember in the design of embedded retaining walls, is the fact that stability is ensured by the capacity of the retaining wall to generated sufficient passive pressure to counter the active pressure on the wall. Generally, the following steps is required to designing an embedded retaining wall:
Site Investigation: A thorough site investigation is the first step in designing an embedded retaining wall. This includes soil testing, groundwater analysis, and assessing the surrounding environment. An understanding of site investigations helps in choosing the appropriate wall type and required design parameters.
Select the Type of Wall: Based on the site conditions and project requirements, select the type of retaining wall. For example, sheet pile walls are often used for temporary structures or where soil conditions allow easy driving. Diaphragm walls are preferred for permanent, deep excavations.
Calculate Earth Pressures: Use soil properties obtained from site investigations to calculate active, passive, and at-rest earth pressures ( Derivation of Action on Retaining Structures to EC7). Make a choice of earth pressure theory (Rankine or Coulomb). For most designs, Rankine’s theory is simpler and is often used for preliminary calculations.
Calculate the Depth of Embedment: The depth of embedment must be sufficient to prevent the wall from rotating or sliding. Use earth pressure calculations and safety factors to determine this depth. Ensure that the embedded portion of the wall can resist both the active earth pressure and any additional loads.
Structural Design: Finally, based on the earth pressures, design the wall to resist the ensuing shear forces and bending moments. For instance, in the case of a reinforced concrete wall, calculate the required wall thickness and reinforce the wall to withstand the bending moments and shear forces exerted by the soil and any external loads.
Worked Example
An embedded retaining wall is required for a construction site to retain soil with a height of 4 meters (Figure 6). The site investigation reveals sandy soil with a unit weight of 18 kN/m³, a soil friction angle of 30 degrees, and no cohesion, indicating it is non-cohesive. Additionally, the water table is below the excavation depth, meaning no hydrostatic pressure needs to be considered in the design.
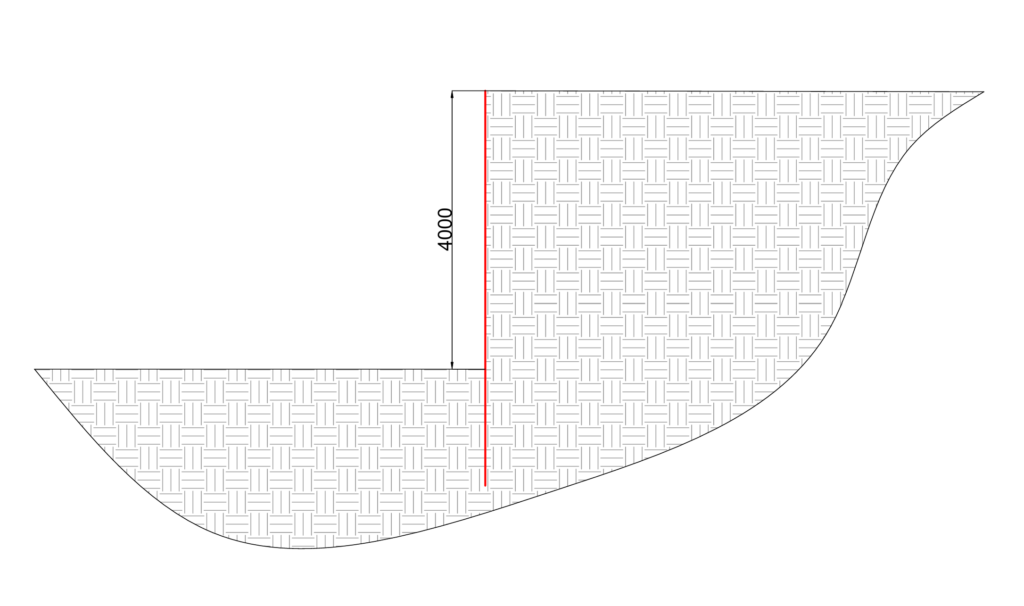
Step 1: Selection of Wall Type
The site investigation reveals a sandy soil with good drainage. The groundwater level is stable, and there are no significant variations in soil properties across the site. Hence, a sheet pile wall is selected due to the sandy soil conditions and the requirement for a relatively shallow excavation.
Step 2: Determination of Earth Pressure
We need to calculate the active and passive earth pressures. The excavation will be 4 meters deep, so we will use this height in our calculations.
First, we calculate the active earth pressure coefficient (Ka) using Rankine’s theory and then use it to determine the active earth pressure:
k_a=\frac{1-sin \phi}{1+sin \phi}=\frac{1-sin30}{1+sin30}=0.33
Next, we calculate the passive earth pressure coefficient (Kp)
k_a=\frac{1+sin \phi}{1-sin \phi}=\frac{1+sin30}{1-sin30}=3.0
Step 4: Active Earth Pressure
Now, we calculate the active earth pressure (Pa) at the base of the excavation (4 meters depth):
\sigma_a=k_a\times \gamma_s \times h\\=0.33\times18\times4 = 24kN/m^2
Step 5: Passive Earth Pressure
Calculate the passive earth pressure (Pp) at the base of the excavation:
\sigma_a=k_p\times \gamma_s \times h\\=3\times18\times4 = 216kN/m^2
Step 6: Determine Depth of Embedment
We use the method of moments to determine the required depth of embedment (D). The moments due to active and passive pressures must balance.
Calculate the moment of active pressure about the toe (point of rotation):
M_a=0.5P_a\frac{h^2}{3}=0.5\times24\times\frac{4^2}{3}=64kN.m/m
Calculate the moment of passive pressure about the toe:
M_p=0.5P_p\frac{d^2}{3}=0.5\times216\times\frac{d^2}{3}=36d^2
For the sheet pile wall to be stable, we set the moments equal
M_a=M_p\\64=36d^2
Solving for d:
d= \sqrt {\frac{64}{36}}= 1.33m
Step 7: Stability Requirements
Stability requires a safety factor for the depth of embedment to account for uncertainties in soil properties and loading conditions. A typical safety factor is 1.5 is generally accepted
d_{design}=1.5\times1.33= 2.0m
The final design requires an embedment depth of 2.0 meters for stability.
Step 8: Check Structural Capacity
The sheet piles must be checked for bending moments and shear forces to ensure they can resist the applied loads. Calculate the maximum bending moment (Mmax) using the triangular distribution of active pressure:
M_{max}= P_a \times \frac{h^2}{8}=\frac{24\times4^2}{8} =48kN.m/m
The chosen sheet pile must have adequate moment resistance to support this bending moment. Select a sheet pile section with a moment capacity greater than 48 kNm/m.
Also See: Designing a Counterfort Retaining Wall | Worked Example
Sources & Citations
- Bowles, J. E. (1996). Foundation analysis and design (5th ed.). McGraw-Hill.
- Das, B. M. (2010). Principles of geotechnical engineering (7th ed.).