This article explores the structural checks many engineers’ neglects. It focuses on areas often bypassed during analysis, design, or detailing.
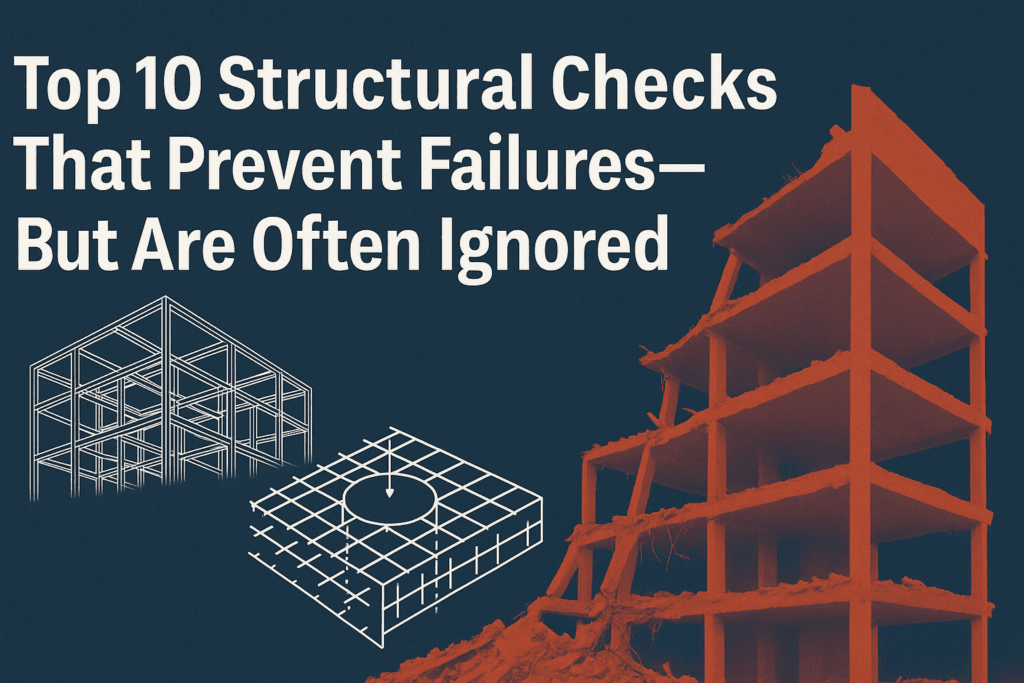
Designing structures involves more than entering values into software and selecting reinforcement. It requires clarity, diligence, and engineering judgment. Structural checks validate how the structure behaves under real-world conditions. Unfortunately, some of the most critical checks remain underappreciated—or completely ignored.
History proves that failure often results from what was not checked. These failures were not caused by a lack of software or code knowledge. They happened because essential verification were missed or assumed. Overlooking key checks does not just reduce safety—it erodes trust in our profession.
This article explores the structural checks many engineers’ neglects. It focuses on areas often bypassed during analysis, design, or detailing. Whether in concrete, steel, or foundation design, these checks can determine the success or collapse of a structure.
Deflection and Serviceability Criteria
Many engineers often meet strength requirements and stop there. But serviceability governs how a structure performs over its life. Deflection structural checks remain the most overlooked, especially when conducting reviews.
Deflections may not affect structural integrity directly, but they can lead to serious functional failures. Excessive sagging can crack finishes, cause drainage issues, or damage partitions. This is particularly problematic in long-span beams, cantilevers, and precast concrete.
In some cases, engineers apply deflection limits without recourse to the time-dependent effects like creep or shrinkage. Creep-induced deflections in prestressed or post-tensioned slabs, if unaccounted for, may lead to severe long-term sagging.
Eurocode 2 and BS 8110 both recommend deflection checks under quasi-permanent loads. Ignoring this check can result in costly post-construction repairs and reduced client confidence and satisfaction with the structure.
Lateral Stability and Frame Sway
Lateral stability checks are often assumed to be covered by the model or simplified through software presets. However, stability can pose to be the weakest link in slender buildings, such as open warehouses, or portal frames.
Neglecting sway structural checks in moment-resisting frames allows uncontrolled horizontal displacement. This can cause cracking in partitions, misalignment of glazing, and even progressive failure under lateral loads.
Designers must check frame behavior under horizontal loads—wind, seismic (where relevant), or accidental. The notional horizontal load method or amplified sway moments (e.g., δ magnification factor in Eurocode) should be assessed.
Sway-sensitive structures must be analyzed explicitly. Frame stiffness, joint rigidity, and diaphragm action all influence stability. Software may calculate displacements, but it is the engineer’s role to assess them against acceptable limits.
Punching Shear in Flat Slabs and Rafts
Flat slabs, mats, and rafts often offer flexibility and fast construction. However, their vulnerability to punching shear is well documented. Engineers sometimes skip these structural checks, especially in early design stages or for smaller column loads.
Punching shear failures are brittle and often sudden. They occur without warning, making them among the most dangerous forms of failure.
Evaluating punching shear at column heads, wall corners, and any point with concentrated loading is important, if these slabs are to be designed properly. Increasing slab thickness, adding shear reinforcement, or using column capitals are mitigation strategies.
Eurocode 2 provides a method for checking shear stress around a critical perimeter. Ignoring this check is especially dangerous in post-tensioned slabs or foundations with uplift forces (See: Designing for Punching in Shear in Concrete Slabs).
Base Plate and Anchor Bolt Design
Structural models often end at the column base. Engineers leave the base plate design for later or assume standard templates. However, base plates and their fixings are fundamental.
Base plate failures have led to collapsed steel frames, especially under combined uplift, shear, and moment. Ignoring plate bearing stress, anchor tension breakout, or anchor bolt edge distances can be catastrophic.
Anchors must be checked for concrete pull-out, steel rupture, and combined tension-shear. The base plate must also be checked for plate bending and grout crushing. These calculations rarely come from the frame model—they require manual checks.
A competent designer must treat base plates as critical elements, not secondary details. They transfer all loads into the foundation and cannot be standardized across varying load conditions.
Torsion in Beams and Edge Elements
Torsion is frequently dismissed unless obviously present. In reality, edge beams, ring beams, and cantilevered components often carry unintended torsional moments due to load eccentricities or continuity.
Ignoring torsion can lead to cracked members and reinforcement misplacement. Structural software may not include torsion effects unless specified manually. Some programs simplify torsional stiffness or ignore it entirely.
Torsion checks should be conducted for:
- Edge beams supporting cantilever slabs
- L-shaped or U-shaped buildings with re-entrant corners
- Horizontal curved beams or ramps
Designers could use Eurocodes torsion design expressions, including combined shear-torsion interaction. Reinforcement must include closed stirrups and longitudinal bars at appropriate locations (See: Designing for Torsion in Steel Elements to Eurocode 3).
Connection Stiffness and Rotational Restraint
Engineers may define joints as pinned or fixed without justification. However, real connections have stiffness that affects structural behavior. Frame analysis without realistic connection properties distorts moment distribution and sway behavior.
Overestimating fixity increases internal forces, while underestimating it under-designs connections. This is particularly serious in semi-continuous steel frames or reinforced concrete beams into columns.
Joint stiffness must be backed by moment-rotation behavior or verified against connection design. Engineers should ensure that assumptions made during global analysis are reflected in the local detailing.
Many failures arise not from member design, but from misunderstood joint behavior.
Load Path Verification
Many engineers rely on software to transfer loads through members, but not every path is direct. Load paths can become complex in multi-level structures, buildings with setbacks, or transfer slabs.
It’s common to find unsupported slab zones, misaligned columns, or discontinuities in transfer elements. These issues aren’t flagged by software unless explicitly modelled to be so.
The engineer must sketch the load path. It should be clear how loads travel from slab to beam to column to foundation. Where discontinuities exist, transfer structures must be verified for bending, shear, and punching.
Misjudging load paths has caused significant structural failures, including during demolition and retrofitting.
Crack Width Control
Again, designing for strength is not enough— for some structures, durability depends on crack control. Engineers often skip crack width checks in reinforced concrete members, especially if they assume minimum cover is sufficient.
Crack widths are defined based on exposure class and structural function. For example, water-retaining structures require tighter limits than typical beams.
Engineers should calculate crack widths using bar spacing, concrete strain, and cover. Overlooking this check can lead to reinforcement corrosion, leakage, and serviceability failures.
Crack control is especially critical in:
- Basement walls
- Tanks and retaining walls
- Slabs with high shrinkage potential
Cracks that go unrestrained can grow wider with time, reducing the design life of the structure.
Second-Order (P-Delta) Effects
Tall, slender, or heavily loaded columns may undergo second-order effects. These are geometric non-linearities that amplify deflections and internal forces.
Software often ignores these unless explicitly requested. Some tools use simplified magnification factors, but engineers must ensure applicability.
Eurocode 2 allows the use of sway/non-sway classification to assess P-Delta relevance. For slender columns, a second-order moment must be added to the primary bending moment.
Neglecting these effects can mean under-designed members that buckle or crack under service loads. Engineers must perform these checks manually if the software cannot.
Foundation Uplift and Settlement
Many engineers design footings based solely on bearing capacity. However, uplift and settlement behaviour are equally critical—especially under wind or moment loading.
Raft foundations under differential loading can tilt. Isolated footings may lift off if tension occurs. These issues are often missed during routine checks.
Engineers must evaluate:
- Foundation bearing pressures under uplift
- Differential settlement between supports
- Uplift under wind for light structures or canopies
Geotechnical data must be consulted, not just for allowable stress, but for compressibility and water table effects. This is important, since foundation design requires interaction between structural and geotechnical inputs.
Conclusion
Structural failures rarely result from one mistake. Instead, they come from a series of missed checks, misunderstood assumptions, and blind trust in automation. Today’s engineer must combine technical skill with judgment.
Structural safety demands more than code compliance. It requires a mindset that questions every assumption, verifies every load path, and treats every detail as critical. This article has outlined ten structural checks, where minor oversights can lead to major failures. Addressing them is not optional—it is the mark of professional responsibility.
The most resilient structures are designed by engineers who check what others assume.
Also See: Common Mistakes Engineers Make in Structural Design and How to Avoid Them
Sources & Citations
- EN 1992-1-1: Eurocode 2: Design of Concrete Structures – General Rules and Rules for Buildings, CEN, 2004.
- The Institution of Structural Engineers (2021). Manual for the Design of Building Structures. IStructE Publications.
- Reynolds, T. & Steedman, J. (2019). Structural Design from First Principles (3rd ed.). Red Globe Press.