This article explores deeply into the core aspects of bridge engineering, explaining the top 9 considerations that sets bridges unique from buildings.
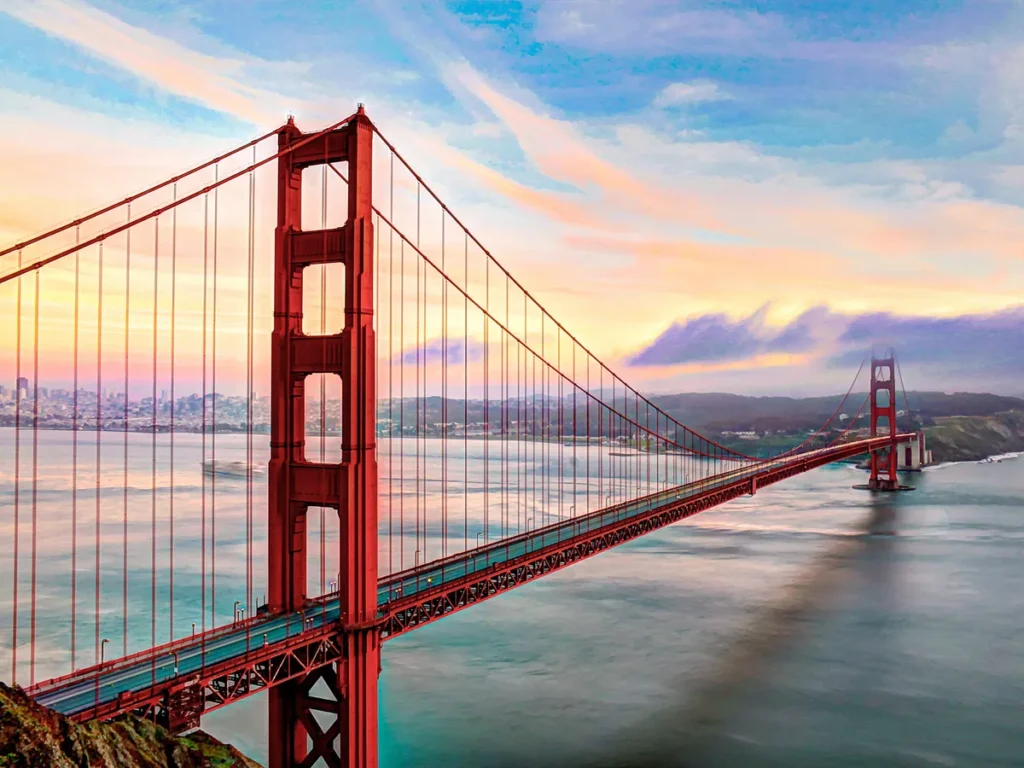
Bridges are far more than functional pathways—they are enduring symbols of human ingenuity and resilience. These structures span rivers, valleys, and cities, bridging not only physical distances but also societal and cultural divides. Their design is a testament to humanity’s pursuit of progress.
However, building a bridge that stands the test of time requires more than just technical knowledge. Bridge design is an art as much as it is a science. It often demands a multidisciplinary approach that integrates construction methodology, environmental considerations, material science, and creative design. Past failures and successes in bridge construction underscore the importance of careful planning, rigorous execution, and innovative thinking.
This article explores deeply into the core aspects of bridge engineering, explaining the top 9 considerations that set bridges unique from buildings. Topics include construction, aesthetics, value, environment, loads, materials, structural elements, effects, and detailing.
Construction: The Foundation of Stability
Unlike buildings, where construction methods might not heavily influence the final structure, bridges demand precision at every stage of construction. The chosen method—whether balanced cantilever, span-by-span, or launching—affects the bridge’s layout, forces, and locked-in stresses. Temporary loads during construction are often greater than operational loads, requiring engineers to meticulously plan each phase.
Each construction method introduces unique challenges. For example, bridges built using launching techniques face forces during erection that differ significantly from those experienced during use. Temporary scaffolding, cranes, or girders interact with the structure, introducing forces that must be carefully managed to avoid long-term damage.
Continuity in design oversight plays a critical role in successful construction. A single guiding hand, such as a lead engineer or team, ensures seamless integration between design and execution. This continuity prevents miscommunication and guarantees that temporary structures and permanent elements align with the broader engineering vision. In the absence of such oversight, inconsistencies may arise, compromising the bridge’s stability and longevity.
Aesthetics: Blending Form with Function
Unlike buildings, where facades often conceal the structural framework, bridges display their engineering openly. This exposure transforms them into works of art as well as feats of engineering. Achieving aesthetic excellence requires balancing form, function, and context, following principles established by Vitruvius: firmitas (durability), utilitas (utility), and venustas (beauty).
Public perception plays a significant role in determining a bridge’s success. Arch bridges, with their sweeping curves, are widely regarded as symbols of strength and elegance. Suspension bridges evoke similar admiration, though their appeal lies in their scale and slenderness. Conversely, beam bridges, while efficient and economical, often lack the visual impact of their counterparts.
The role of architects in bridge design remains a subject of debate. While their involvement can enhance visual harmony, the ultimate responsibility for aesthetics rests with the engineer. Bridges designed with structural logic tend to possess an inherent elegance. For example, bridges that emphasize the natural flow of forces—such as catenary or arch structures—often achieve beauty through their functionality.
Value: Maximizing Efficiency and Impact
Bridge projects, unlike buildings, are heavily dominated by structural costs. While a building’s structural content may account for 20% of its total cost, in bridges, this figure often exceeds 90%. As a result, engineers play a leading role in balancing costs, durability, and design excellence.
Value in bridge engineering extends beyond construction expenses. It encompasses long-term maintenance, environmental impact, and societal benefits. Publicly funded bridges, in particular, must demonstrate exceptional utility and durability to justify their expense. Innovations in construction techniques, such as prefabrication and modularity, often provide cost-effective solutions without compromising quality.
Speed of construction is another critical factor. Faster construction reduces costs associated with traffic management and site occupation. This is particularly relevant in urban settings, where prolonged disruptions can have cascading economic effects. In rural areas, mechanization can help reduce labor costs and accelerate timelines.
Environment: Weathering the Elements
Bridges face relentless environmental pressures. Unlike buildings, which are often shielded by cladding, bridges are directly exposed to sun, rain, and wind. These forces demand careful consideration of materials, drainage systems, and thermal expansion mechanisms.
Temperature changes cause axial movements of up to ±400mm in longer bridges, necessitating the use of expansion joints and flexible bearings. Integral bridge designs, which eliminate bearings and rely on structural flexibility, are often preferred for spans under 60 meters. For longer spans, fixed and guided bearings are essential to accommodate movements while resisting lateral and longitudinal forces.
Rain and salt-laden water pose additional challenges, particularly in regions where de-icing salts are used. Corrosion-resistant materials, combined with effective drainage systems, help mitigate these risks. Advances in concrete technology, such as the use of fly ash and ground granulated blast-furnace slag (GGBS), have improved the long-term durability of bridge decks and supports.
Loads: Designing for Strength and Resilience
The loads a bridge must bear far exceed those of typical buildings. Traffic loads, particularly from heavy vehicles, are concentrated and dynamic, requiring detailed analysis and robust structural solutions. Bridges must also account for lateral and longitudinal forces, such as wind, braking, and centrifugal effects.
Finite-element analysis (FEA) and influence line techniques are invaluable in understanding load distribution. These tools help engineers identify peak forces and optimize structural elements to withstand them. While traditional methods like line beams and 2D grillages suffice for simpler designs, complex bridges often require 3D modeling to account for torsional effects and dynamic interactions.
Bridges also face unique challenges in reducing self-weight. Innovative designs, such as orthotropic steel decks and profiled concrete slabs, minimize material use without sacrificing strength. These approaches are particularly important for longer spans, where self-weight becomes a dominant factor in design.
Materials: Balancing Strength and Innovation
Material selection plays a pivotal role in bridge design. Reinforced concrete (RC) and prestressed concrete (PSC) dominate in medium-length spans, while steel and composite materials are preferred for longer spans. Each material offers unique advantages, shaped by site conditions, budget, and performance requirements.
PSC, in particular, transforms concrete into a versatile material capable of withstanding tension. Prestressing techniques—whether internal or external—allow for bespoke designs that maximize efficiency. Steel, with its superior tensile strength, remains indispensable for longer spans and intricate geometries. Hybrid systems, which combine concrete and steel, are increasingly popular for their ability to balance compression and tension forces effectively.
Sustainability is an emerging priority in material selection. Weathering steel, which forms a protective patina, and eco-friendly concrete mixes demonstrate the industry’s shift toward reducing environmental impact and maintenance demands.
Structural Elements: Optimizing Design
Bridge spans, which often exceed 100 meters, demand structural elements far larger and more complex than those in buildings. Key components include deck slabs, girders, and piers, each tailored to the bridge’s specific demands.
Deck slabs, typically 250mm thick, must resist concentrated wheel loads while maintaining a lightweight profile. Composite designs, where concrete slabs interact with steel girders via shear studs, enhance load distribution and stiffness. Girders, whether I-shaped or box-shaped, vary in thickness and reinforcement to balance local and global stresses.
Piers, often constructed from reinforced concrete, provide the vertical support necessary for stability. Their design considers not only axial loads but also lateral and impact forces, particularly in seismic zones or regions prone to vehicular collisions.
Structural Effects: Beyond the Basics
Bridges must account for structural effects often ignored in buildings. These include shear lag, temperature differentials, and creep, all of which influence long-term performance.
Shear lag, for instance, reduces the effective width of deck slabs and girders, concentrating stresses near webs. Temperature differentials create locked-in stresses, with warmer surfaces expanding more than cooler cores. Creep, particularly in prestressed concrete, causes gradual shifts in load distribution, necessitating careful analysis during design and construction.
Detailing: The Devil in the Details
Attention to detail is paramount in bridge design. Poorly designed joints, inadequate reinforcement, or sub-optimal drainage can undermine an otherwise robust structure. Detailing encompasses everything from reinforcement placement to welding specifications, ensuring each element contributes to the bridge’s overall stability.
Technological advancements, such as Building Information Modeling (BIM), have revolutionized detailing by enabling precise visualizations and integrations. However, the role of experienced engineers remains critical. They must translate complex forces into practical solutions, balancing safety, aesthetics, and constructability.
Conclusion
Bridges are more than just functional structures; they are feats of engineering and design that embody both creativity and technical precision. Unlike buildings, which often conceal their inner workings, bridges lay their design open for the world to see, making their structural integrity and aesthetic value inextricably linked. The unique challenges of managing loads, choosing materials, and adapting to the environment require engineers to think differently and more innovatively.
Also See: Construction of Concrete Bridges -Selecting a Bridge Layout
Sources and Citations
- Concrete Bridge Development Group (2014). Concrete Bridge Design and Construction Series: Best Construction Methods for Concrete Bridges. The Structural Engineer.
- Bourne, S. (2019). An Introduction to Bridges for Structural Engineers (Part 1). The Structural Engineer, January 2019.
- Bourne, S. (2019). An Introduction to Bridges for Structural Engineers (Part 2). The Structural Engineer, March 2019.
- Steel Construction Institute (2015). SCI Publication P185: Guidance Notes on Best Practice in Steel Bridge Construction.
- Vitruvius, P. (30 BCE). De Architectura.