This article explores human–structure interaction from the structural engineering perspective.
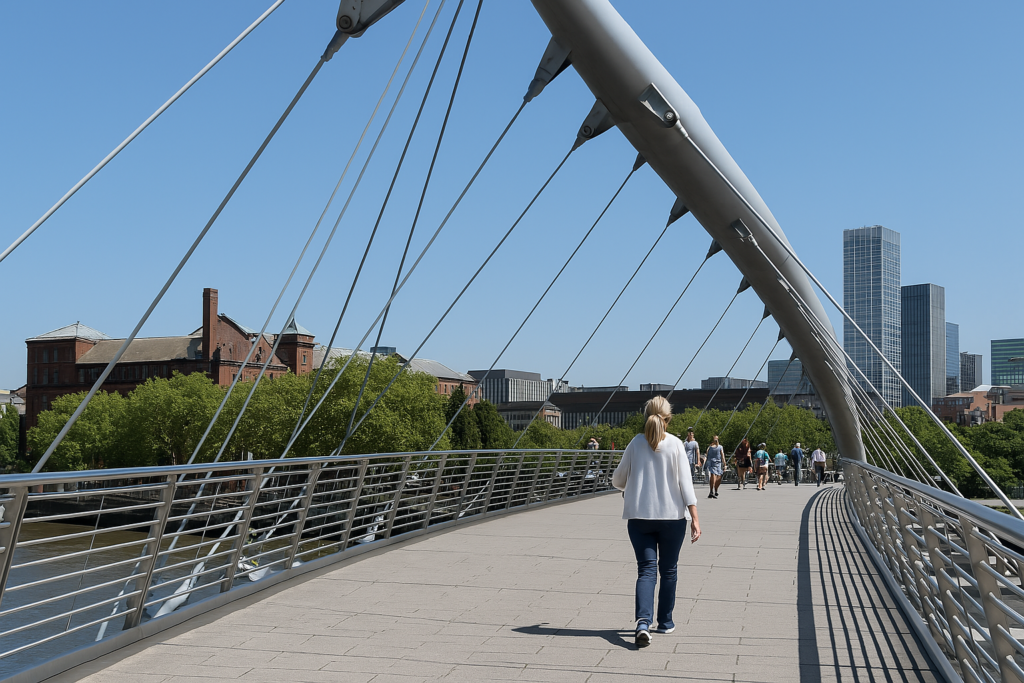
Have you ever wondered why a bridge vibrates slightly under your feet or why a stadium sways during a cheer? These reactions aren’t flaws. They reveal a critical concept in structural engineering: human–structure interaction. As structures become lighter and spans grow longer, this interaction becomes more noticeable and increasingly important.
Modern design emphasizes efficiency, flexibility, and economy. Engineers reduce material use by creating slender members and expansive floors. These features enhance aesthetics and sustainability. But they also introduce dynamic behavior. When people walk, jump, or gather, they induce forces. Structures respond. Understanding this interaction matters in both safety and comfort.
This article explores human–structure interaction from the structural engineering perspective. It examine its mechanics, identify key parameters, analyze practical implications, and explain how engineers predict, control, and design for it. This phenomenon blends physics, perception, and design intuition. Understanding it allows engineers to create buildings and infrastructure that serve and satisfy users in real-world conditions.
Understanding Human–Structure Interaction
Human–structure interaction refers to the feedback loop between human-induced forces and a structure’s dynamic response. It differs from static load scenarios, where people act as stationary weights. In dynamic contexts, people become active participants. They generate vibrations through movement. The structure responds, often influencing human motion in return.
This feedback can either dampen or amplify structural vibrations. For instance, a pedestrian walking across a footbridge produces rhythmic loading. If the bridge’s natural frequency matches the pace of walking, resonance may occur. This creates visible motion. In some cases, the motion causes discomfort or even fear, despite structural safety.
The interaction intensifies with crowd behavior. A single person rarely induces noticeable vibration. But crowds, especially moving in sync, can excite the structure significantly. In stadiums, arenas, or busy walkways, this interaction requires careful consideration during design.
Key Parameters Influencing the Interaction
Several parameters determine the nature and magnitude of human–structure interaction. The most significant include the mass of the structure, its stiffness, natural frequencies, damping ratio, and human movement characteristics. Each contributes uniquely to the overall behavior.
Mass and stiffness define how easily a structure moves under force. Heavier and stiffer structures resist movement, while lighter and more flexible ones respond more. Natural frequency determines how readily the structure responds to certain rhythmic forces. If the force frequency matches the natural frequency, resonance occurs.
Damping measures how quickly the structure returns to rest after being disturbed. Low damping allows vibrations to persist. High damping absorbs energy quickly. Human motion also varies widely. Walking, running, jumping, or dancing each generate different loading patterns. Step frequency, stride length, and synchronization across groups affect the total response.
Human-Induced Loads and Structural Vibrations
Human motion applies dynamic loads. Engineers often characterize them by frequency and amplitude. A walking person imposes a load with a typical frequency of 1.6–2.2 Hz. Running increases this to 2.5–3.5 Hz. Jumping induces even higher frequencies and sharper impacts.
Structures possess their own natural frequencies. If these align with human-induced loads, significant motion can occur. A common example is the vibration felt on lightweight pedestrian bridges. In extreme cases, this causes perceptible vertical and lateral motion. A well-known case occurred with the London Millennium Bridge, which swayed laterally due to synchronized pedestrian steps on its opening day.
These phenomena are not due to structural weakness. Instead, they result from dynamic amplification. In the Millennium Bridge’s case, pedestrians unconsciously adjusted their gait to the bridge’s movement. This synchronization increased the load frequency, reinforcing the motion.
Vibration Perception and Comfort Criteria
Structural engineers must address not only strength and stability but also user comfort. Vibrations that remain within safe limits may still cause unease or discomfort. Human sensitivity to vibration varies by frequency, amplitude, and direction.
Vertical vibrations are more tolerable than lateral ones. Occupants in offices or residential spaces typically notice vibrations exceeding 0.5% of gravitational acceleration (0.005g). In contrast, public footbridges can allow higher levels, depending on context.
Design codes and guidelines specify acceptable vibration limits. These include ISO 10137, SCI P354 (for floors), and Eurocode EN 1990 Annexe A1. Engineers compare calculated structural responses against these criteria. If the response exceeds thresholds, damping enhancement or structural stiffening may be required.
Modelling Human–Structure Interaction
Engineers use analytical and numerical tools to model human–structure interaction. These include simplified dynamic models and detailed finite element simulations. The simplest model treats the human as a moving harmonic load. More advanced methods incorporate biomechanical properties of the human body.
Some models treat humans as mass-spring-damper systems. These models can simulate the transfer of energy between people and structure. Others include walking models with force-time histories derived from experiments. These models improve prediction accuracy, especially for crowded conditions.
Crowd behaviour introduces another layer of complexity. Engineers use stochastic models to simulate random footfall patterns or apply experimental data from motion capture studies. The goal is to replicate real-world conditions and predict the resulting vibrations.
Strategies to Control Undesirable Vibrations
When analysis indicates problematic human–structure interaction, engineers explore control strategies. They aim to reduce vibration amplitude, shift natural frequencies, or increase damping. These strategies include structural modifications, passive devices, and tuned systems.
Increasing stiffness raises the natural frequency. Engineers achieve this by adding cross-bracing, deepening beams, or reducing span lengths. However, this increases cost and material use. Enhancing damping is often more efficient. Materials like viscoelastic polymers or additional non-structural components can absorb energy.
Tuned mass dampers (TMDs) are widely used in sensitive structures. A TMD consists of a secondary mass attached with springs and dampers. It moves out of phase with the structure, canceling vibration energy. This method is common in high-rise buildings and long-span bridges.
Other methods include tuned liquid dampers, base isolators, or human feedback suppression strategies. In some cases, adjusting usage patterns or controlling access can mitigate issues. For example, restricting simultaneous crowd movement on footbridges avoids synchronization.
Design Applications and Practical Considerations
Human–structure interaction affects multiple structure types. Footbridges, floors in offices, stadium stands, and concert venues all experience dynamic loading from people. Engineers must account for it from concept to final design.
In footbridges, the primary concern is lateral vibration. Engineers must consider not only vertical forces but also torsional and sideways motion. Floor vibration in offices can arise from people walking or machinery. Lightweight floor systems demand extra care to maintain comfort standards.
In stadiums, the synchronized movement of large crowds generates complex vibration patterns. Engineers must design to prevent amplification of rhythmic motion. Concert halls require attention to both structural and acoustic vibration. Materials and structural geometry must balance comfort and sound quality.
Designers often test prototypes or mock-ups before construction. These include scale models or full-scale vibration testing. These experiments validate assumptions and guide modifications.
Building Codes and Guidelines
Various codes offer guidance on human–structure interaction. They provide acceptable vibration thresholds and methods for calculating structural response. Some of the most referenced documents include:
- ISO 10137: Basis for assessing serviceability due to human-induced vibration.
- SCI P354: Guidance for steel-framed floor vibrations in offices and schools.
- Eurocode EN 1990 Annex A1: Partial safety factors and serviceability limits.
- TRADA and Arup Guidelines: Used for timber structures and specialist cases.
While codes offer numeric criteria, they also rely on engineering judgment. Each structure is unique. Engineers must combine code compliance with contextual understanding of the structure’s use, form, and materials.
Trends and Research Directions
Research continues to expand the understanding of human–structure interaction. Recent work focuses on real-time motion capture, artificial intelligence, and machine learning. These tools improve load predictions and vibration analysis.
Sensor networks embedded in structures now provide real-time feedback. These data help monitor vibration levels and adjust usage if needed. For example, smart footbridges can issue alerts or modify lighting to influence walking patterns.
Biomechanical studies refine human movement models. As researchers understand the variability of human gait and behavior, structural responses can be predicted with greater accuracy.
There is growing interest in interaction not only between humans and structures but between crowds and flexible infrastructure. Engineers aim to design spaces that dynamically adapt to user movement. These adaptive structures may use actuators or shape-memory materials to respond in real time.
Conclusion
Human–structure interaction bridges engineering, comfort, and perception. It emerges from the dynamic relationship between people and their built environment. As structures become lighter and more flexible, this interaction becomes increasingly critical.
Engineers must understand how humans load structures. They must also predict how structures respond and ensure that these responses stay within safe and comfortable limits. Achieving this requires dynamic analysis, experimental validation, and practical design measures.
Also See: Soil Structure Interaction: A Comprehensive Overview
Sources & Citations
- ISO 10137. (2007). Bases for design of structures – Serviceability of buildings and walkways against vibrations.
- Smith, A. L., Hicks, S. J., & Devine, P. J. (2007). Design of floors for vibration: A new approach. SCI Publication P354.
- Zivanovic, S., Pavic, A., & Reynolds, P. (2005). Vibration serviceability of footbridges under human-induced excitation: A literature review. Journal of Sound and Vibration, 279(1–2), 1–74.
- Brownjohn, J. M. W. (2007). Footbridge crowd dynamics: Theory, measurement, and design. Structural Engineering International, 17(2), 108–112.