The Eight article in the series on design and construction of concrete bridges, explores the subject of prestressing as it concerns concrete bridges.
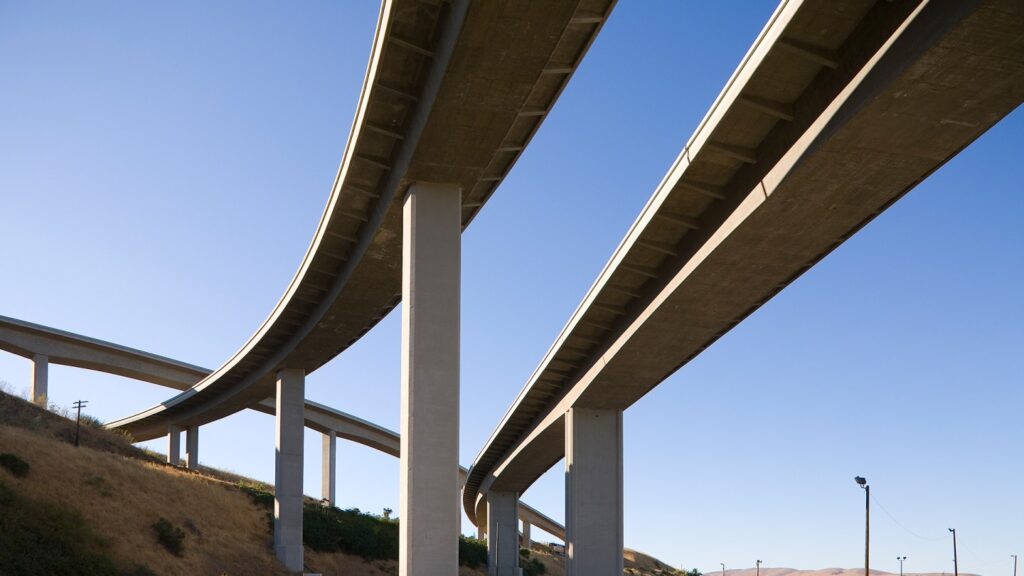
Prestressing plays a crucial role in the construction of concrete bridges. It enhances the performance and efficiency of concrete members, particularly in structures like bridges that must handle significant loads and stresses. Traditionally, concrete is strong in compression but weak in tension. Prestressing effectively strengthens concrete in both respects, allowing it to carry loads more efficiently and making the construction of lighter, more economical bridges possible.
The importance of prestressing cannot be understated when it comes to concrete bridges, particularly for spans greater than 20 to 30 meters. Without prestressing, these structures would require larger, heavier components to resist tension forces, increasing costs and affecting the aesthetic design. Prestressing introduces internal forces that counterbalance the tension stresses from external loads, allowing bridges to be built with slender and elegant members that maintain high durability and resistance to cracking. This minimizes long-term maintenance needs, making the overall project more cost-effective.
Furthermore, prestressing significantly impacts on steel congestion within the concrete, allowing for easier and quicker construction. As prestressed sections remain compressed under permanent loads, they behave more elastically and with increased stiffness. This not only simplifies the control of deflections but also enhances the overall stability and longevity of the structure. In short, prestressing enables engineers to overcome the inherent limitations of concrete, making it an indispensable element in the design and construction of modern concrete bridges.
Fundamentals of Prestressing
Prestressing is an active process where internal forces are applied to counteract the external loads that a structure will face throughout its life. This is achieved by applying tension to steel tendons or cables embedded in the concrete. The tension in these cables induces compression in the surrounding concrete, thereby increasing its ability to resist external forces. This makes prestressed concrete stronger in both tension and compression, allowing for greater flexibility in design and construction.
For a concrete bridge, understanding the mechanics of prestressing is essential for both the design and the construction phases. A bridge deck typically consists of various slabs, webs, and supports (Figure 1). The top slabs are governed by traffic loads and bending effects, while the webs are responsible for shear and torsion at supports. Designers must carefully consider the effects of these loads when planning the layout of prestressing cables. Additionally, keeping the overall weight of the concrete to a minimum is vital, as self-weight dominates many bridge designs.
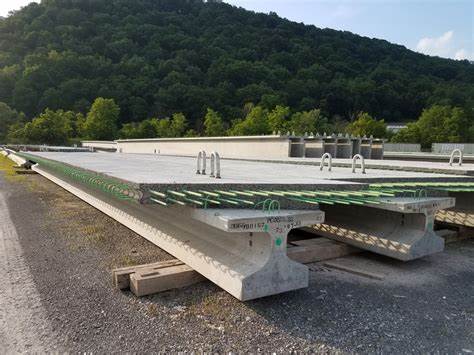
Prestressed sections are also compressed under permanent effects, providing additional stiffness and durability. These sections behave elastically under loads, allowing for more efficient control of deflections. Designers must also consider the “kern height” of the section, which defines the area within which prestressing forces can be applied without causing the concrete to go into tension (Figure 2). A higher Kern efficiency, typically around 60% for box girders or I-beams, ensures that the prestress is effectively distributed throughout the section, reducing material wastage and improving the overall performance of the bridge.
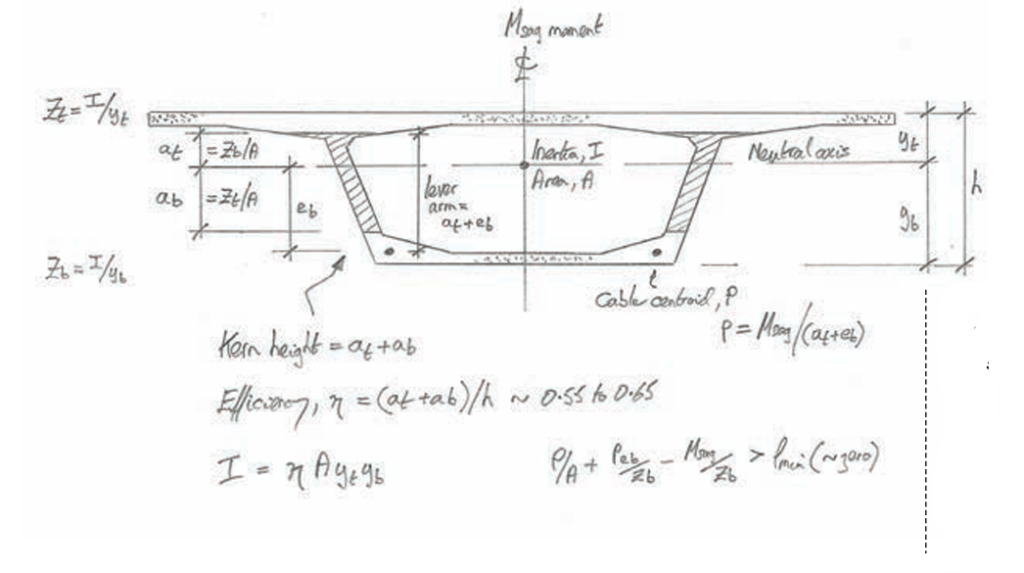
Pre-stressing in Concrete Bridge Design
When designing concrete bridges, the placement and layout of prestressing cables are crucial to ensuring the bridge’s strength and durability. The positioning of these cables is determined based on the moments (bending forces) acting on the structure. In areas where the bridge experiences sagging moments, prestressing cables are placed towards the bottom of the concrete section. In contrast, they are positioned closer to the top where hogging moments (upward bending forces) occur. By carefully analyzing these moments and placing the prestressing cables accordingly, engineers can ensure that the bridge remains fully compressed, even under maximum loading conditions.
In continuous bridges, prestressing introduces both primary and secondary moments. The primary moments are caused directly by the prestressing force itself, while secondary moments result from the interaction between the bridge’s structural elements and the prestress. These secondary moments, often referred to as parasitic moments, are particularly significant in multi-span bridges, where they can help to redistribute forces between supports and mid-span sections. By carefully managing these moments, engineers can fine-tune the prestress to achieve an optimal balance between strength and flexibility.
One of the key advantages of prestressing is its ability to reduce the amount of reinforcing steel required in a concrete structure. Thus, applying pre-compression to the concrete, prestressing minimizes the need for additional steel reinforcement, which simplifies the construction process and reduces costs. This is particularly important in regions where space constraints make it difficult to place large amounts of reinforcement. In bridge design, minimizing steel congestion allows for easier placement of concrete, improving the quality of the final structure.
Pre-tensioned and Post-tensioned Bridges
Prestressing can be applied in two ways: pre-tensioning and post-tensioning. In pre-tensioned bridges, the steel tendons are tensioned before the concrete is poured (Figure 3). Once the concrete has hardened, the tendons are released, transferring the tension to the concrete and introducing compression. This method is typically used for precast beams and smaller bridges, where the design calls for straight, uniform sections.
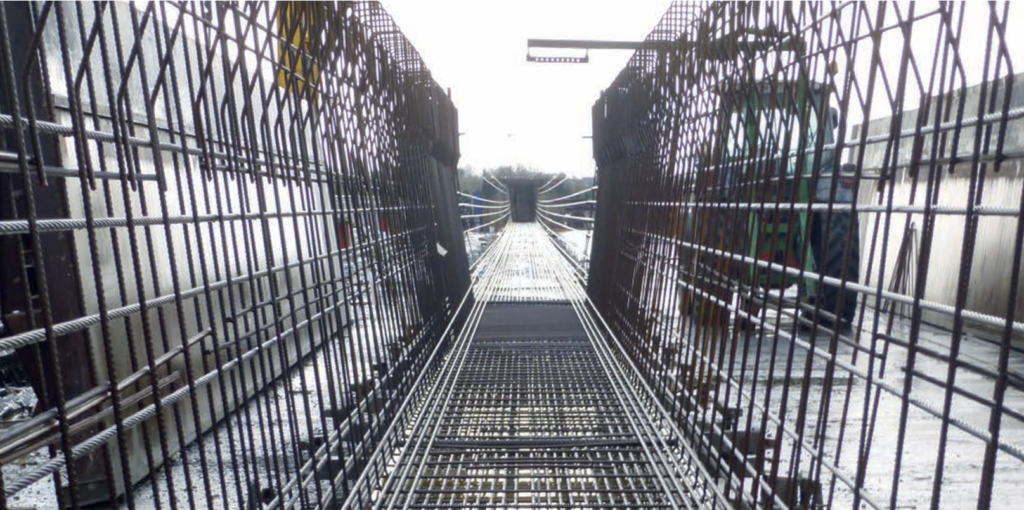
Pre-tensioned concrete beams are often manufactured off-site in precast factories and transported to the construction site. This method has several advantages, including higher quality control and faster construction times. Pre-tensioned beams are commonly used for spans ranging from 5 to 40 meters and can be quickly erected using cranes. They are particularly useful for projects where minimal traffic disruption is required, such as bridges over busy highways or railways.
On the other hand, post-tensioning involves casting the concrete first and then tensioning the steel tendons after the concrete has cured (Figure 4). This method is more versatile than pre-tensioning, as it allows for more complex shapes and longer spans. Post-tensioned bridges can accommodate spans of up to 300 meters, making them ideal for large-scale infrastructure projects. The tendons in post-tensioned bridges are placed inside ducts within the concrete, and after tensioning, the ducts are filled with grout to protect the steel from corrosion.
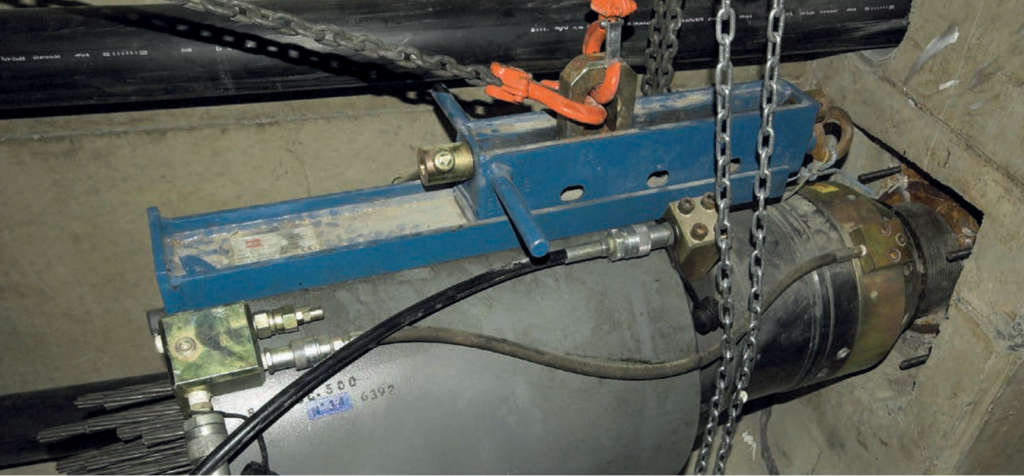
External and Internal Pre-stressing
Post-tensioning can be further divided into internal and external pre-stressing, depending on where the tendons are placed. Internal pre-stressing involves placing the tendons inside the concrete section, where they are fully bonded to the concrete through the grouting process (Figure 5). Internal pre-stressing provides greater structural efficiency, as the tendons can be placed in the exact locations needed to counteract bending moments. This method is commonly used in box girders and I-beams, where the tendons are positioned along the top and bottom slabs to resist sagging and hogging moments.
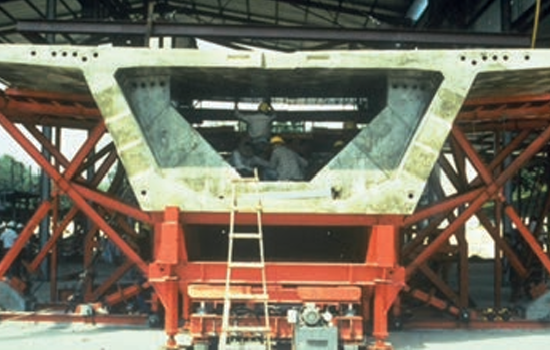
External pre-stressing, on the other hand, involves placing the tendons outside the concrete section, typically within ducts made of high-density polyethylene (HDPE) (Figure 6). External pre-stressing is often used in larger bridges, where the simplicity of installation and maintenance outweighs the slight reduction in structural efficiency. One of the key advantages of external pre-stressing is that the tendons can be easily inspected and replaced, if necessary, which is particularly important for long-span bridges that may require periodic maintenance over their service life.
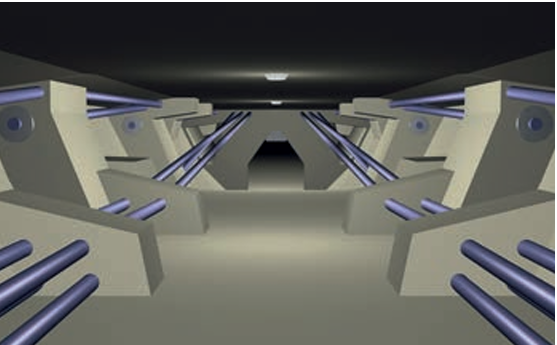
In modern bridge design, a combination of internal and external pre-stressing is often used to achieve the best of both worlds. Internal tendons provide the necessary structural strength and durability, while external tendons allow for easier inspection and maintenance. A careful balance of both systems allows engineers to design bridges that are both efficient and long-lasting.
Durability and Long-Term Performance
One of the main benefits of pre-stressing in concrete bridges is its ability to enhance the long-term durability of the structure. By keeping the concrete under compression, pre-stressing reduces the likelihood of cracking, which is a primary cause of deterioration in concrete bridges. Cracks allow water and other corrosive elements to penetrate the concrete, leading to the corrosion of reinforcing steel and the eventual weakening of the structure. Pre-stressing minimizes this risk by maintaining compression in the concrete, even under heavy traffic loads.
Additionally, pre-stressing allows for the use of higher-strength concrete, which further improves the bridge’s resistance to environmental factors such as freeze-thaw cycles, chemical exposure, and wear and tear from heavy vehicles. This makes pre-stressed concrete bridges particularly well-suited for harsh environments, where traditional concrete structures may suffer from premature degradation.
The long-term performance of a pre-stressed bridge is also enhanced by the careful control of the pre-stressing forces. Over time, factors such as creep, shrinkage, and relaxation can cause the pre-stress to decrease, which can lead to increased deflections and a reduction in the overall strength of the structure. By accounting for these factors during the design phase and regularly monitoring the bridge’s performance, engineers can ensure that the pre-stressing system continues to function as intended throughout the bridge’s service life.
Also See: Designing a Post-tensioned Flat Slab | Worked Example
Conclusion
Pre-stressing is a vital component in the design and construction of concrete bridges. It enhances the material’s strength in both compression and tension, allowing for the creation of lighter, more economical structures that can span greater distances while maintaining high levels of durability and performance. Whether through pre-tensioning or post-tensioning, the careful application of pre-stressing forces ensures that concrete bridges remain stable and resilient, even under the most demanding conditions.
Sources & Citations
- Benaim, R. (2008). The Design of Prestressed Concrete Bridges: Concepts and Principles. Taylor & Francis.
- Menn, C. (1990). Prestressed Concrete Bridges. Birkhauser.
- Concrete Bridge Development Group (CBDG). (2014). Prestressing for Concrete Bridges. The Structural Engineer, 92(3), 48-52.