This article examines the design and construction techniques for concrete arches, frames, tunnels, and jacked boxes, covering a complete spectrum of bridge types, from the smallest culverts to the world’s longest concrete spans
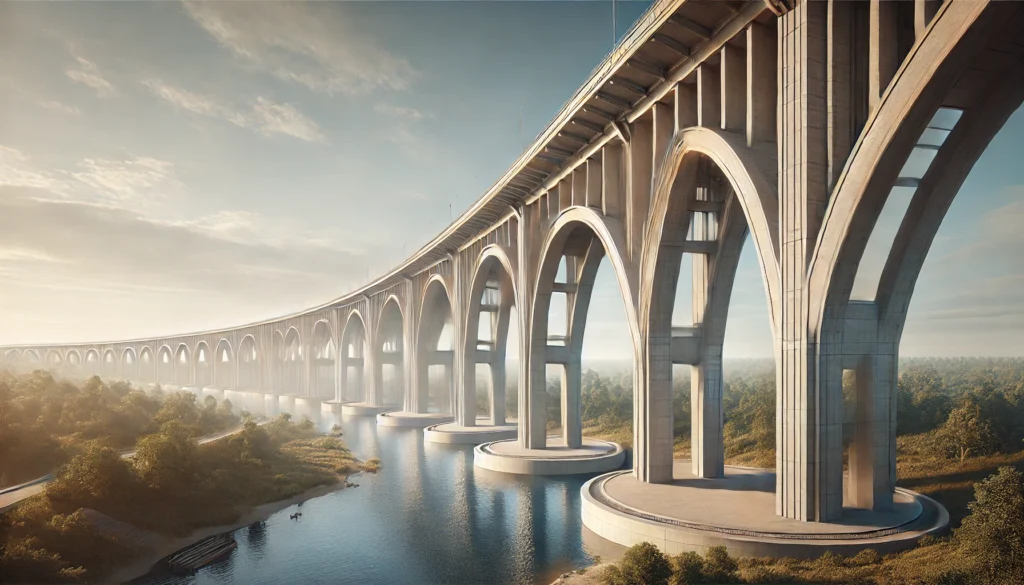
Concrete bridge construction encompasses diverse methods suited for different environments and load requirements. This article examines the design and construction techniques for concrete arches, frames, tunnels, and jacked boxes, covering a complete spectrum of bridge types, from the smallest culverts to the world’s longest concrete spans. These methods are essential in creating functional connections across roads, railways, and pedestrian paths, handling varied spans and load-bearing demands.
Concrete arches, frames, and tunnel solutions have become essential for modern infrastructure. Each type offers distinct benefits: arches provide durability and compression efficiency, frames offer a hybrid approach to load management, and tunnels ensure minimal disruption in crowded spaces. The article references construction techniques in both standard and bespoke configurations, demonstrating how these methods have adapted to the needs of different site locations and project demands.
The methods discussed here, illustrated by images from various real-world projects, provide insights into construction practices for bridges serving critical transit routes, waterways, and other infrastructures. From rapid assembly methods for urban areas to large-span bespoke structures for expansive terrain, concrete bridges are central to infrastructure networks worldwide.
Arch Bridges
Concrete arch bridges are efficient structures that employ axial compression to handle load transfer to foundations. Two main types are prevalent: standard precast arches and bespoke arches. Standard precast arches, as shown in Figure 1, are typically suited for spans between 5 and 25 meters. These arches are often used in earth-retaining spans and are efficient due to their ability to distribute traffic and fill loads with minimal bending forces. The structural design considers span-to-rise ratios of 2 to 3, depending on overburden and ground conditions.
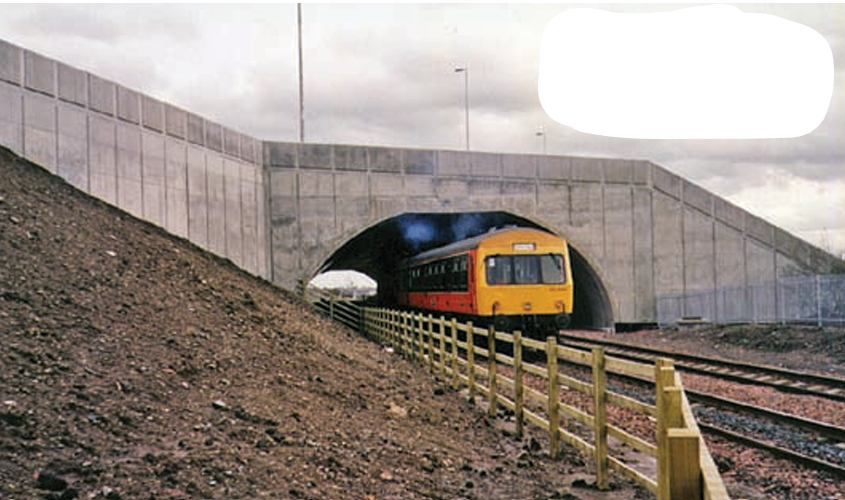
Bespoke arches on the other hand are tailored for larger spans, commonly ranging from 50 to 400 meters. These customized arch bridges are adapted to unique site needs and conditions, often requiring a single large span and reliable foundations. Their structural form varies to manage load distribution, with curvature adjustments that optimize clearance and stability. Bespoke arches are exemplified by the Bloukrans Bridge in South Africa (Figure 2), a major bridge with a 272-meter main span that balances aesthetic appeal with compression efficiency. These longer arch bridges serve as both functional structures and visual landmarks, reflecting engineering expertise and precision.
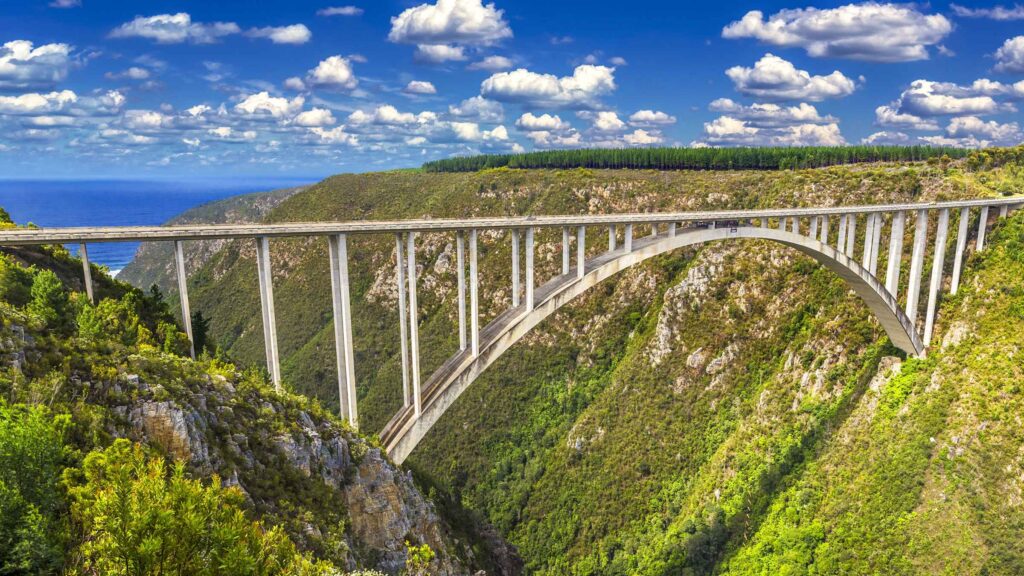
Frame Bridges
Frame bridges combine the benefits of beam and arch designs, bearing loads through a mix of bending and compression. With legs and a polygonal intrados instead of a typical arch curve, frame bridges efficiently distribute loads, making them suitable for various terrain and location constraints. The River Tyne Bridge in the UK (Figure 3) exemplifies a frame bridge designed to accommodate large spans while preserving aesthetic appeal. Frame bridges are usually cast in situ, allowing for structural adjustments that meet site-specific needs.
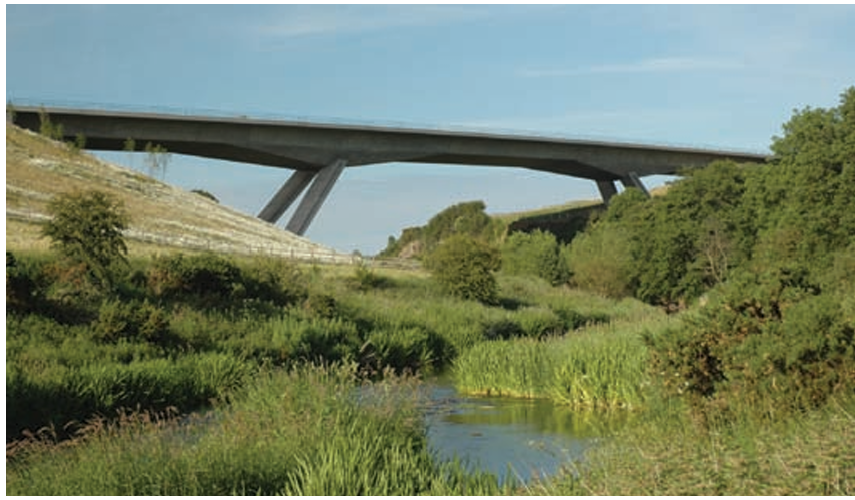
Frame bridges are versatile and often serve areas where both beam and arch designs might apply, as they manage complex load paths with aesthetic flexibility. Their use of legs and decks allows frame bridges to support more intricate structural patterns, making them suitable for unique sites with significant span requirements. Due to their adaptability, frame bridges are a popular choice in environments with difficult terrain or visual restrictions.
Tunnel Bridges and Jacked Portals
Tunnel and jacked portal bridges offer solutions where conventional bridge erection would disrupt traffic flow or surrounding infrastructure. For tunnels, cut-and-cover methods or jacked portals, which allow entire bridge sections to be prefabricated and then positioned under existing infrastructure. For instance, the A43 bridge in Northamptonshire (Figure 4) uses a jacked box design that minimizes disruption by pushing prefabricated concrete sections into place.
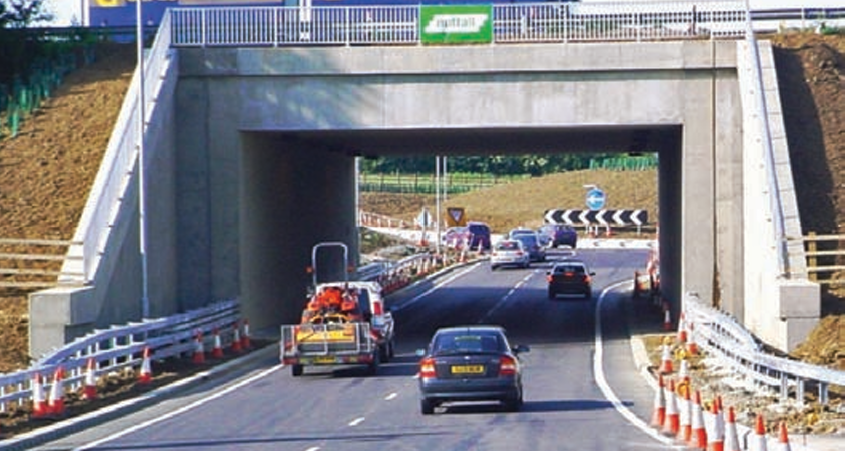
Cut-and-cover tunnels are built by excavating a trench for the structure, which is then backfilled after completion. This method is effective for locations with ample space for excavation and provides a durable, underground passage. Standard precast culvert units (Figure 5) are common in smaller tunnels, spanning up to 6 meters. These tunnels are suitable for small waterways, pedestrian underpasses, and minor vehicle access points, offering an efficient solution for limited-span needs.
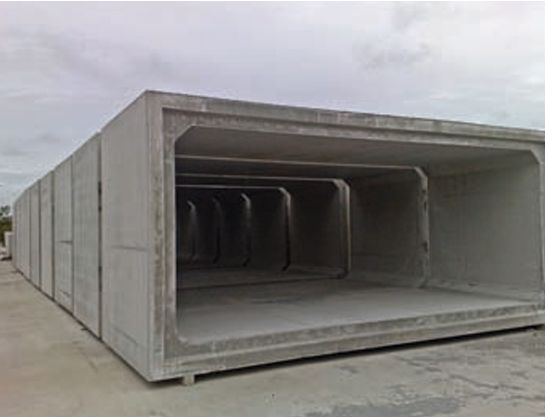
Jacked portals, or box structures, are especially advantageous in busy urban areas where traffic cannot be significantly disrupted. In these cases, the structure is prefabricated in a nearby location and moved into place using slide tracks or hydraulic jacks. This method allows bridge construction to occur with minimal on-site interference, reducing construction timelines and associated risks.
Construction Techniques
Construction of the various types of bridges can happen using any of the following methods, as expatiated in the next sections.
Precast Construction
Precast construction allows components for arches, frames, and tunnels to be manufactured off-site. These components are then assembled on-site, as seen with precast arch units in Figure 6. Precast arches are typically assembled with socket details at their bearing points to manage stress concentrations. This approach not only expedites construction but also enhances quality control by allowing sections to be produced in factory conditions.
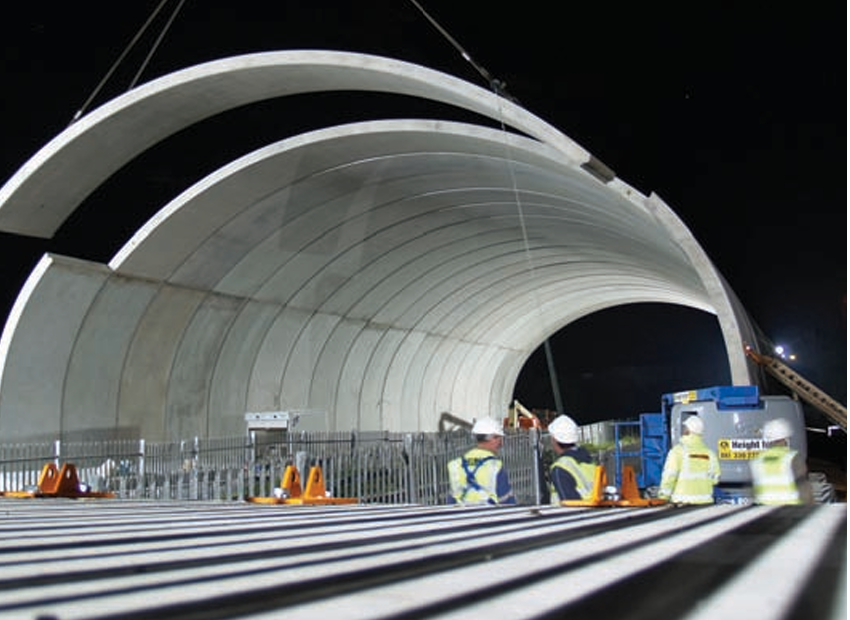
Precast box culverts also support efficient construction in tunnel systems. These culverts are often connected on-site, with waterproofing applied at joints to ensure stability and prevent water intrusion. As exemplified in Figure 5, these units are commonly used for small tunnels and access routes. This method is advantageous in urban areas, where construction speed and minimal disturbance are priorities.
In Situ Construction
In situ construction is common for frame bridges, allowing structures to be cast directly at the construction site. This method is preferred when a project requires specific adjustments or when the terrain does not support extensive pre-casting. For example, frame bridge legs and decks, as seen in Figure 6, are often constructed in situ to fit unique site requirements and load conditions. In situ construction also allows for structural flexibility, making it easier to adapt bridge sections to varied environments.
In some cases, frame bridges may require temporary supports or scaffolding to hold sections in place while concrete sets. This method ensures that the structure conforms to precise dimensions and material specifications while retaining the option for mid-construction adjustments based on site conditions.
Advanced Erection Techniques
Innovative erection techniques streamline the bridge construction process, particularly for larger spans. Methods like balanced cantilevering and rotational erection are used to efficiently assemble large structures without extensive temporary works. Balanced cantilevering, illustrated by the Sungai Dinding Bridge in Malaysia, involves casting segments incrementally from each end, meeting at the center. This method is ideal for constructing arches over deep valleys or water, where supporting the structure from below is impractical.
In rotational erection, bridge sections are cast beside or above the final location and then rotated into place. This approach, used in projects like Ove Arup’s Kingsgate Footbridge in Durham, UK, minimizes groundwork and enables precision placement. These methods are effective for both arch and frame bridges, providing alternatives that limit site disturbances and improve construction timelines.
Structural Considerations
Load Management and Stability
Concrete bridges must be designed to manage various load types, including traffic, environmental pressures, and self-weight. Arch and frame bridges distribute loads through axial compression and bending. For instance, arch bridges like the Maryville Railway Bridge in the UK (Figure 1) transfer loads through compression, reducing stress on other structural elements. Frame bridges, by contrast, handle complex load paths due to their polygonal design, ensuring even weight distribution across the structure.
Tunnel and jacked portal bridges are subject to significant horizontal earth pressures, necessitating careful geotechnical analysis to ensure stability. For these structures, waterproofing and soil interaction are critical considerations. Design adjustments account for potential movement and pressure shifts due to soil composition and water levels, ensuring the bridge structure remains stable over time.
Material and Environmental Durability
Concrete’s resilience and strength make it suitable for enduring structures, but each project may require material customisations. For example, some arches may use polymeric fabrics or waterproof coatings to extend their lifespan in areas with high moisture or variable temperatures. Concrete mix variations and reinforcement are also applied to improve durability and manage cracking. Waterproofing, especially around joints, is crucial to protecting concrete from environmental factors.
For aesthetically prominent bridges, the appearance of concrete also contributes to design choices. Bespoke arches, like the Bloukrans Bridge (Figure 3), balance visual appeal with structural efficiency, while demonstrating how concrete can be both functional and elegant. These bridges are often designed as structures that harmonize with the surrounding environment while meeting the rigorous structural demands.
Aesthetic and Environmental Impact
Many bespoke bridge designs emphasize both functionality and aesthetic appeal. For example, Robert Maillart’s Salginatobel Bridge in Switzerland showcases the careful attention to detail in arch and deck junctions, creating a visually pleasing yet structurally robust design. Meanwhile, tunnel bridges and jacked portals minimize visible impact by remaining largely underground, preserving the natural or urban environment while providing essential transit routes.
Conclusion
Standard and bespoke arches, frame bridges, and tunnel solutions each serve specific structural functions, from urban underpasses to large, landmark spans over rivers and valleys. As illustrated in the examples from this article, these concrete bridges support infrastructure networks that meet both functional and environmental goals.
Also See: Design of Concrete Bridges – Understanding Prestressing
Sources and Citations
- Concrete Bridge Development Group. (2014). Concrete Bridge Design and Construction series No.8: Concrete bridge construction methods – arches and frames. The Structural Engineer, 92(8), 34-38.
- Benaim, R. (2008). The Design of Prestressed Concrete Bridges: Concepts and Principles. Taylor & Francis.
- Institution of Structural Engineers. (2004). Design and Construction of Deep Basements including Cut-and-Cover Tunnels. London, UK: Institution of Structural Engineers.