This article explores the fundamentals of carbon in structures, discusses the methods used to calculate embodied carbon, and provides a fully worked example of calculating the carbon footprint of a reinforced concrete slab.
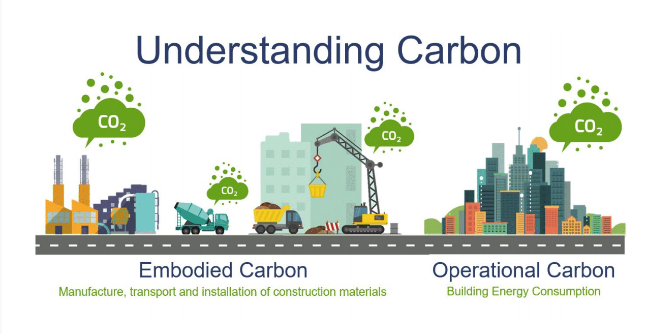
The construction industry produces nearly 40% of global carbon emissions. Embodied carbon comes from material production, transportation, and construction. Manufacturing processes release significant carbon dioxide (CO₂), increasing the environmental impact. Structural engineers and designers must reduce these emissions by selecting sustainable materials and improving construction methods. Optimizing material use lowers carbon footprints and enhances sustainability. As environmental concerns grow, engineers play a crucial role in creating low-carbon structures through informed design and construction choices.
Carbon emissions in structures arise from multiple sources, including the extraction and processing of raw materials, energy consumption during manufacturing, and transportation to the construction site. Each stage of a building’s life cycle contributes to its overall carbon footprint, making it necessary to assess and mitigate emissions at every step. Steel, concrete, and timber are the primary materials used in structural design, each with a distinct carbon profile. The selection of materials, along with sustainable design practices, significantly impacts a structure’s environmental performance.
Understanding carbon footprints calculations is essential for engineers seeking to design sustainable structures. The ability to quantify and compare emissions from different materials allows for better decision-making in structural design. This article explores the fundamentals of carbon in structures, discusses the methods used to calculate embodied carbon, and provides a fully worked example of calculating the carbon footprint of a reinforced concrete slab.
Understanding Carbon in Structures
Carbon emissions in structures can be categorized into two main types: embodied carbon and operational carbon. Embodied carbon refers to the CO₂ emitted throughout the material life cycle, starting from raw material extraction, transportation, and manufacturing to installation, maintenance, and eventual demolition. Once materials are produced and used in a structure, their embodied carbon remains fixed. On the other hand, operational carbon is associated with energy consumption during a building’s use, including heating, cooling, lighting, and electrical systems. While energy efficiency measures can reduce operational carbon over time, embodied carbon must be minimized at the design and construction stages.
The materials used in structural engineering have a substantial impact on a building’s embodied carbon. Concrete, being the most widely used construction material, has a high carbon footprint due to the energy-intensive production of cement. The chemical process of cement production releases a large amount of CO₂, making it a major contributor to construction-related emissions. Steel, commonly used in high-rise buildings and bridges, also has a high embodied carbon due to the energy required for its extraction and refining. In contrast, timber is considered a low-carbon material because it absorbs CO₂ during growth, making it a more sustainable option when sourced from responsibly managed forests.
Apart from material selection, transportation distance and construction techniques also affect the carbon footprint of a structure. Locally sourced materials reduce transportation-related emissions, while efficient construction methods can optimize material usage and reduce waste. Engineers and designers must consider these factors when calculating a building’s total carbon footprints, ensuring that every stage of the construction process aligns with sustainability goals.
Methods of Calculating Carbon in Structures
Carbon calculation in structures uses various methods to assess emissions at different life cycle stages. Life-Cycle Assessment (LCA) evaluates environmental impacts from material extraction to disposal. Cradle-to-gate covers emissions up to manufacturing. Cradle-to-grave includes production, use, demolition, and waste disposal. Cradle-to-cradle promotes recycling and reuse, reducing raw material demand.
To perform accurate carbon calculations, engineers rely on standardized carbon factors, which quantify the amount of CO₂ emitted per unit of material. These values are obtained from databases such as the Inventory of Carbon and Energy (ICE) and Environmental Product Declarations (EPD). The ICE database provides embodied carbon values for commonly used construction materials, allowing engineers to estimate emissions based on material quantities. EPDs, on the other hand, offer manufacturer-specific carbon data, giving a more precise assessment of material footprints.
The formula used to calculate embodied carbon in structures is based on material quantities and their corresponding carbon factors. The total embodied carbon of a structure is determined using the equation:
EC =\Sigma(M_i \times CF_i)
where EC represents the total embodied carbon (measured in kgCO₂e). Mᵢ denotes the mass or volume of material. i, and CFᵢ is the carbon factor of material i (expressed in kgCO₂e per kg or cubic meter). We apply this equation to quantify and compare the carbon footprints of different structural designs.
Worked Example: Calculating Carbon in a Reinforced Concrete Slab
Consider a reinforced concrete slab with dimensions 8 meters by 6 meters by 0.2 meters. The slab is made of C30/37 concrete, with a density of 2400 kg/m³. And a carbon factor of 0.15 kgCO₂e per kg. Additionally, the slab contains reinforcement steel at a rate of 80 kg per cubic meter of concrete. A carbon factor of 2.50 kgCO₂e per kg. The objective is to calculate the total embodied carbon of the slab.
Step 1: Calculate the Volume and Mass of Concrete
The volume of the concrete slab is determined by multiplying its length, width, and thickness:
V_{conc}=8\times8\times0.2=9.6m^3
Using the density of concrete, the total mass is calculated as follows:
M_{conc}=9.6\times2400=23,040kg
Step 2: Calculate the Embodied Carbon of Concrete
Applying the carbon factor for concrete:
EC_{conc}=M_{conc}\times CF_{conc}
23,040kg\times0.15kgCO_2e/kg =3456kgCO_2e
Step 3: Calculate the Mass of Reinforcement Steel
Since reinforcement steel is provided at 80 kg per cubic meter of concrete, the total mass of steel is:
M_{steel}=V_{conc}\times 80
=9.6\times80 =768kg
Step 4: Calculate the Embodied Carbon of Reinforcement Steel
Using the carbon factor for steel:
EC_{steel}=M_{steel}\times CF_{steel}
768kg\times2.50kgCO_2e/kg =1920kgCO_2e
Step 5: Determine the Total Embodied Carbon of the Slab
Summing up the embodied carbon contributions from concrete and steel:
EC_{total}=EC_{conc}+EC_{steel}
=3456+1920=5376kgCO_2e
The reinforced concrete slab has an embodied carbon of 5,376 kgCO₂e. Reducing this footprint requires strategic material choices. Using fly ash or ground granulated blast-furnace slag lowers cement emissions. Selecting recycled steel cuts reinforcement-related carbon. Optimizing slab thickness minimizes material use. These adjustments significantly reduce environmental impact and support sustainable construction.
Conclusion
Calculating a structure’s carbon footprint ensures sustainable construction. Engineers must reduce embodied and operational carbon when designing buildings. Material selection and construction methods significantly impact emissions. Life-cycle assessments and carbon factor databases help in making informed, low-carbon decisions. The worked example shows how to apply carbon calculations in practice. Sustainable material choices remain essential in structural engineering. As the industry moves toward net-zero, accurate carbon accounting will shape construction’s future.
Also See: Estimating the Embodied Carbon in Structures
Sources and Citations
- Hammond, G., & Jones, C. (2011). Inventory of Carbon & Energy (ICE). University of Bath.
- The Institution of Structural Engineers. (2020). How to Calculate Embodied Carbon.
- British Standards Institution. (2019). BS EN 15978: Sustainability of Construction Works – Assessment of Environmental Performance of Buildings.
- International Energy Agency (IEA). (2021). Net-Zero by 2050: A Roadmap for the Global Energy Sector.