This article explores the principles behind robustness checks, their significance, and why treating them as just a code requirement is not enough.
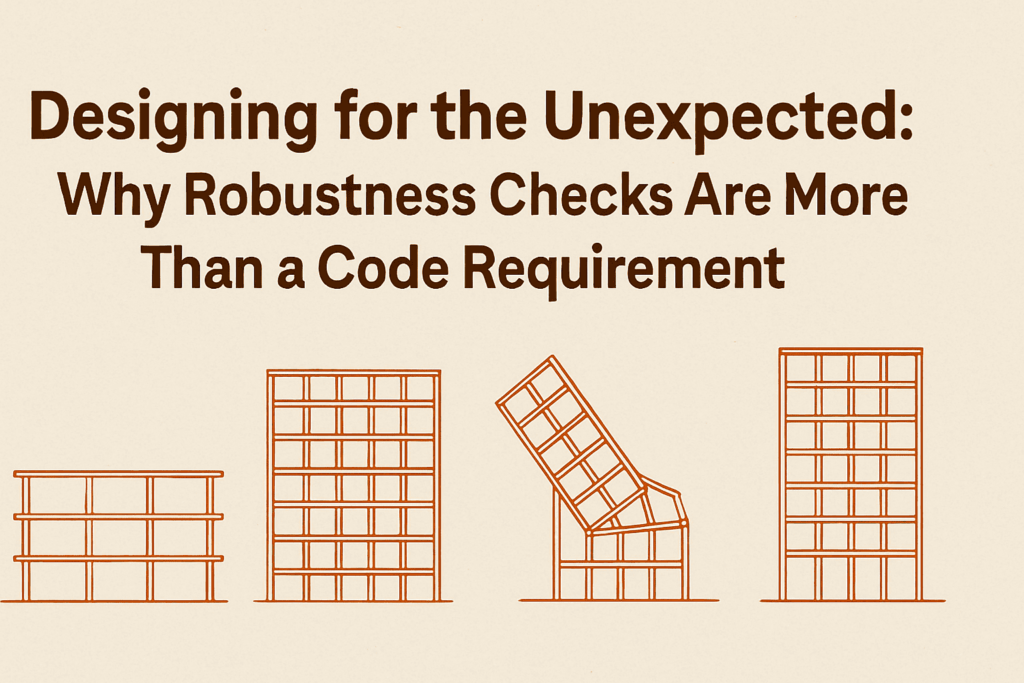
Modern buildings face risks that go far beyond the typical design loads. From unforeseen explosions to sudden impacts and progressive collapses, real-life events often expose hidden weaknesses in structures. While building codes provide minimum design standards, they cannot account for every possible failure scenario. This is where robustness checks come in—offering a layer of assurance that extends beyond the conventional design routines.
Robustness is not a new idea. Historical collapses such as the Ronan Point disaster in 1968 and more recent tragedies like the Surfside condominium collapse in 2021 have underscored the cost of overlooking systemic vulnerabilities. These events show that failure is not always due to poor design but often due to lack of redundancy, ductility, and awareness of how a local failure might propagate. Designing for robustness is about preparing for these worst-case scenarios.
This article explores the principles behind robustness checks, their significance, and why treating them as just a code requirement is not enough.
Understanding Structural Robustness
Structural robustness refers to a building’s ability to withstand events that were not explicitly considered in its original design. A robust structure resists disproportionate collapse. It localizes damage rather than allowing it to cascade into a full-system failure.
This means that when one part of a structure fails, the rest can continue to stand—at least long enough to prevent loss of life. Robustness is rooted in principles of redundancy, continuity, and ductility. Redundancy means alternate load paths exist. Continuity ensures members work together under stress. Ductility allows elements to deform without snapping.
Most codes, including Eurocode EN 1991-1-7 and ASCE 7-22, require designers to consider accidental actions such as explosions, impacts, or internal failures. However, the real challenge lies in how designers interpret and apply these requirements. Some view robustness checks as another tick-box activity. But in practice, they demand critical thinking and engineering judgment.
Why Code Compliance Isn’t Enough
Codes often times just set minimum thresholds. They aim to create a baseline for public safety, but not to guarantee resilience under every circumstance. Real-world performance, however, depends on how well engineers go beyond these thresholds.
Many robustness provisions in codes are vague. For example, Eurocode permits either a risk-based approach, a prescriptive tie-force method, or an alternative load-path method. But it does not explicitly define how far to go with each. This vagueness can lead to under-design or superficial compliance. Engineers may follow the tie-force method without truly examining load redistribution paths or connection behaviours during failure.
Real-life failures highlight this gap. In 1981, the Hyatt Regency walkway in Kansas City collapsed because of a connection detail change. The structure met code requirement, but it lacked robustness. Similarly, the 1995 Sampoong Department Store collapse in South Korea involved a change in usage and unauthorized structural alterations. The building technically met minimum design loads, but its poor ductility and lack of redundancy made failure inevitable once the system was disturbed.
Robustness is a mindset, not a formula. It means looking at the structure as a system, not just a collection of elements. It requires asking, “What happens if this fails?” and tracing the consequences throughout the structure. Thus, codes are just a starting point, not the finish line.
The Progressive Collapse Problem
Progressive collapse, also called disproportionate collapse, occurs when a local failure triggers a chain reaction, bringing down much of the structure. The initial trigger may be small—an explosion, impact, or structural modification—but its effects escalate due to lack of arrest mechanisms in the system.
The Ronan Point collapse in London is one of the earliest wake-up calls. In 1968, a gas explosion blew out a precast panel on the 18th floor. The panels were load-bearing and poorly tied together. The loss of one panel led to a vertical collapse of an entire corner of the building.
Progressive collapse happens when:
- Load paths are not redundant.
- Structural continuity is weak.
- Connections are brittle.
- There is a lack of compartmentalization.
A very good designer can prevent it by applying principles of robustness early in design. This includes checking for alternate load paths, introducing continuous ties, and verifying that critical elements can resist abnormal loads. Analytical tools such as nonlinear dynamic simulations and pushdown analyses are invaluable in identifying failure chains before construction begins.
Beyond the Tie-Force Method
The tie-force method, popularized in codes like Eurocode EN 1991-1-7, mandates that structural elements (especially in buildings with concrete or steel frames) include continuous ties—horizontal and vertical. These ties prevent parts of the structure from separating under accidental actions.
While effective for uniform structures, this method has limitations. It assumes that the ties will always act as intended. But in reality, construction errors, detailing flaws, or material degradation can undermine tie performance. The method also may not capture complex load redistributions that occur after element failure.
A more reliable approach involves alternative load path analysis. This method removes a critical element in a structural model and examines how loads redistribute. If the structure can still carry the loads through different paths without collapse, it passes the robustness test. This approach, though more demanding, offers better insight into an actual system behaviour.
Dynamic nonlinear analysis goes a step further. It simulates the real-time progression of damage and collapse, capturing inertia, damping, and plastic deformation. These tools are especially useful in performance-based design and high-risk structures like stadiums, bridges, and tall buildings.
Case Studies in Robust Design
1. 7 World Trade Center, New York (2006)
Rebuilt after 9/11, this building incorporates advanced robustness features. Designers used transfer trusses, redundant frames, and fire-resistant materials. Nonlinear analyses validated alternate load paths for extreme events. The building’s design philosophy embraced worst-case scenarios, not just typical load cases.
2. The Pentagon Renovation (Completed before 9/11)
Ironically, the renovated portion of the Pentagon was the least damaged during the 9/11 attack. This section had upgraded connections and improved ductility. The robustness features slowed down the collapse, giving occupants time to evacuate.
3. Christchurch Justice and Emergency Services Precinct, New Zealand
Designed for post-earthquake functionality, this facility used base isolation, ductile detailing, and rigorous redundancy checks. The design anticipated structural and non-structural failures and planned for continued operation after major seismic events.
These projects show that robust design does not just prevent collapse. It protects occupants, while preserving function.
Tools and Techniques for Robustness Analysis
Robustness analysis requires tools that go beyond elastic models. Nonlinear static and dynamic tools are essential. These include:
- Pushdown analysis: Gradually increases gravity load on a damaged structure to study redistribution.
- Time-history analysis: Simulates structural behaviour over time under dynamic loads or removals.
- Component-level analysis: Tests critical joints and connections under extreme deformation.
- Progressive collapse software: Packages like SAP2000, ETABS, and LS-DYNA offer advanced modelling for failure mechanisms.
Equally important is scenario-based modelling. Engineers must create and analyse realistic abnormal event scenarios—such as vehicle impact, gas explosion, or column removal. This shift thinking from idealized conditions to possible disasters.
In physical terms, robustness also means:
- Detailing for ductility and energy absorption.
- Using fire-resistant materials.
- Designing with compartmentalization to limit damage spread.
Integrating Robustness into Design Thinking
Robustness should be an early-stage consideration—not a late-stage add-on. It should inform structural layout, material choices, and connection details. Designers must ask: how would this structure behave if something goes wrong?
Coordination between disciplines is also key. Architects, structural engineers, and MEP professionals must work together to ensure system-wide robustness. A well-placed shear wall is useless if penetrated by services that weaken it. Fire can spread if there are no fire stops. Collaboration builds robust buildings.
Risk-based design is another approach. It focuses on consequences and probabilities. For high-risk buildings like hospitals, airports, or nuclear plants, the cost of failure is too great. Here, robustness becomes a design priority, not an option.
Ultimately, robust design is part of ethical engineering. It prioritizes safety, resilience, and foresight. It acknowledges uncertainty, and not just compliance.
Conclusion
Robustness checks are more than a code requirement. They are an ethical obligation and a technical necessity. Structural engineers must prepare for scenarios where things go wrong. A well-designed building doesn’t just stand up—it refuses to fall down without a fight. Designing for robustness means thinking systemically, testing assumptions, and simulating failure. It means embracing uncertainty and building structures that are not just functional, but resilient. It calls for courage to go beyond the minimum and design for the unknown.
Also See: Structural Redundancy and Progressive Collapse Prevention
Sources & Citations
- Eurocode 1: Actions on Structures – Part 1-7: Accidental Actions (EN 1991-1-7). European Committee for Standardization (CEN), 2006.
- ASCE/SEI 7-22: Minimum Design Loads and Associated Criteria for Buildings and Other Structures. American Society of Civil Engineers (ASCE), 2022.
- Starossek, U., & Haberland, M. (2011). “Disproportionate Collapse: Terminology and Procedure.” Engineering Structures, 33(11), 2801–2806.
- DoD UFC 4-023-03: Design of Buildings to Resist Progressive Collapse. U.S. Department of Defense Unified Facilities Criteria, 2018.